Introduction
In her rise and progress Chemistry presents a remarkable page in the history of science. While no other field of human knowledge remained so long untilled, no other ever presented such glorious results of flower and fruit, after the first germ had taken root.
The purely abstract sciences, philosophy, and especially mathematics, find their origin in a hoary antiquity, and their earliest teachings may serve as means wherewith to form the youthful mind of the present generation; they soon attained a rank among the true sciences, and what they taught has been but more firmly established by the lapse of ages. Not so with the natural sciences. It is not easy to assign the reason why the ancient naturalists, with all their acuteness, should have permitted their strivings after truth to be frustrated by an almost utter neglect of observation. Yet we cannot deny that efforts were made, with respect to many departments of science, to establish them on a firm basis. Aristotle divided the natural world into three great classes. His views of matter, however, as those of the other Grecian philosophers, could not stand a moment after chemistry became a science. Anaximander taught four elements as the primary constituents of our planet, fire, air, water, and earth; and centuries after no alteration was made in the doctrine, though the economical application of many substances, and the manipulation of many natural products, offered chemical facts which might have led the way to a scientific appreciation of chemical combinations and decompositions. The ancient Egyptians prepared many salts, as sal ammoniac, carbonate of soda, sulphate of iron, as also glass and tiles; they were able to reduce many metals from their ores, and to make various alloys. The embalming of dead bodies, the preparation of medicines, the fabrication of vinegar, beer, and other artificial products, as practised by the Egyptians, presuppose some chemical experience. The knowledge possessed by the Egyptians with respect to these and many other subjects, was diffused at a later period among the Jews and Greeks, and perhaps among the Chinese also. Yet, notwithstanding all this, we cannot go beyond the end of the seventeenth century, for the origin of scientific chemistry.
The manner in which chemistry at this time commenced her career is exceedingly remarkable. It was not the effort to elucidate certain obscure ideas with respect to the combinations of matter, it was a delusion which occupied men's minds for many centuries in succession; and in futile endeavors to find the philosopher's stone and the elixir of life, a mass of facts was accumulated which served as material for the foundations of chemistry. From the seventh to the eleventh century the Arabians were principally engaged in the discovery and preparation of medicines. In a complete ignorance of the true character of the mineral kingdom, it is exceedingly likely that the problem of finding a substance which should heal all diseases, and transmute a base metal into a noble one, was suggested by the fact of their finding occasionally in the ashes of some worthless earth, or as the result of some chance experiment, a highly valued metal. The substance to which the above-mentioned virtues were ascribed was called the philosopher’s stone, and the endeavor to find it caused, first of all, the Arabians to make a host of combinations of the most different substances. In this way they made many discoveries which were carried to Europe during the crusades, where the endeavor to transmute the common metals into gold was prosecuted with unprecedented ardor. From the thirteenth to the seventeenth century the art of making gold attained the rank of a so-called science, and while there were so many persons who actually did hope to solve the problem, this art, alchemy, gained the great consideration which it at that time enjoyed by means of deceivers, who knew how to conceal finely comminuted gold in substances, which, when mixed with lead, became visible for the first time, appearing as if an actual transmutation of the baser metal into gold had taken place. The writings of the alchemists were preserved as treasures, and they had at least the result of teaching a number of observations on the affinities of different substances, which, when properly understood, greatly facilitated the rapid progress of chemistry. The first chemical system arose with George Ernest Stahl, towards the beginning of the eighteenth century, a system which endeavored to arrange all known facts according to general principles; thus the development of chemistry falls within the limits of the preceding and the present century.
In its present form chemistry is the science of matter. It teaches the properties of matter, its mutual relations, and the laws of its combinations. These are ascertained in two ways: by combining several elements in a given manner, or by separating combinations into their elements, and measuring or weighing the ingredients. The latter method, or analysis, is by far more productive in results than the former, or synthesis. To recompose a substance from its elements, after these have been accurately ascertained, is a problem of great difficulty in many cases.
The number of simple substances contained in our earth is not very great, as we shall see hereafter, but the combinations of several single elements are numerous, while the secondary combinations with one another of these primary combinations of elements are vastly greater in amount, new ones being almost daily added in the progress of science. It is only necessary to point the observer to the various minerals, plants, and animals, of which each one possesses some peculiar odor, or color, or taste, or product, or property, each involving some chemical difference, to give him some idea of how numerous the various combinations of elementary matter must be. The same consideration will also suggest how varied must be the experience to be collected by chemistry, and how diversified the experiments by which she ascertains her facts. With the progress of any science, the amount of its elucidatory and accessory apparatus increases, the latter being the true exponent of the former. In this view, a description of chemical apparatus, and of its application, will give us an idea of the present state of the great science of modern chemistry.
Conditions of Aggregation of Matter
All ponderable matter, whether belonging to the mineral, to the vegetable, or to the animal kingdom, presents itself to us under one of three conditions of aggregation, depending on the temperature and pressure to which the body may be subjected. These states of matter are the solid, the liquid, and the gaseous.
The four elements of Anaximander, earth, water, air, fire, illustrate the difference between the idea of an element as entertained by the ancients and by the modern philosopher. The elements of the former, instead of expressing the elementary simple ingredients of which all bodies are composed, merely typified the three states of matter, earth answering to the solid, water to the liquid, and air to the gaseous. The fourth element, fire, may be considered as symbolizing the effects of the imponderable agents, heat, light, magnetism, and electricity.
We may safely assume that all solids are capable of being changed into liquids and gases, by the application of the proper agencies. It is well known that many of these solids are capable of this transformation, as seen in the conversion of the solid lump of ice, by the simple application of heat, into the liquid, water, and finally into the gas. steam. The fact, however, is not so generally appreciated that gold may be vaporized by the focal heat of a large mirror, and that the apparently infusible platinum, the metal which can withstand the furnace seven times heated, is speedily made to boil and disappear by the flame of the oxy -hydrogen blowpipe. We may therefore assume that all solids are capable of these transformations by the increase of heat and diminution of pressure. The converse is also most probably true, to the same extent, that all gaseous matter may be converted to solid by the diminution of heat and increase of pressure. In many cases, either heat or pressure, applied positively or negatively, may suffice; in others, both are required; and if they produce the desired effect neither singly nor in combination, we may analogically conclude that it is because of the inadequacy of our means, and not of the impossibility of the end.
Apparatus for Melting Solid Bodies
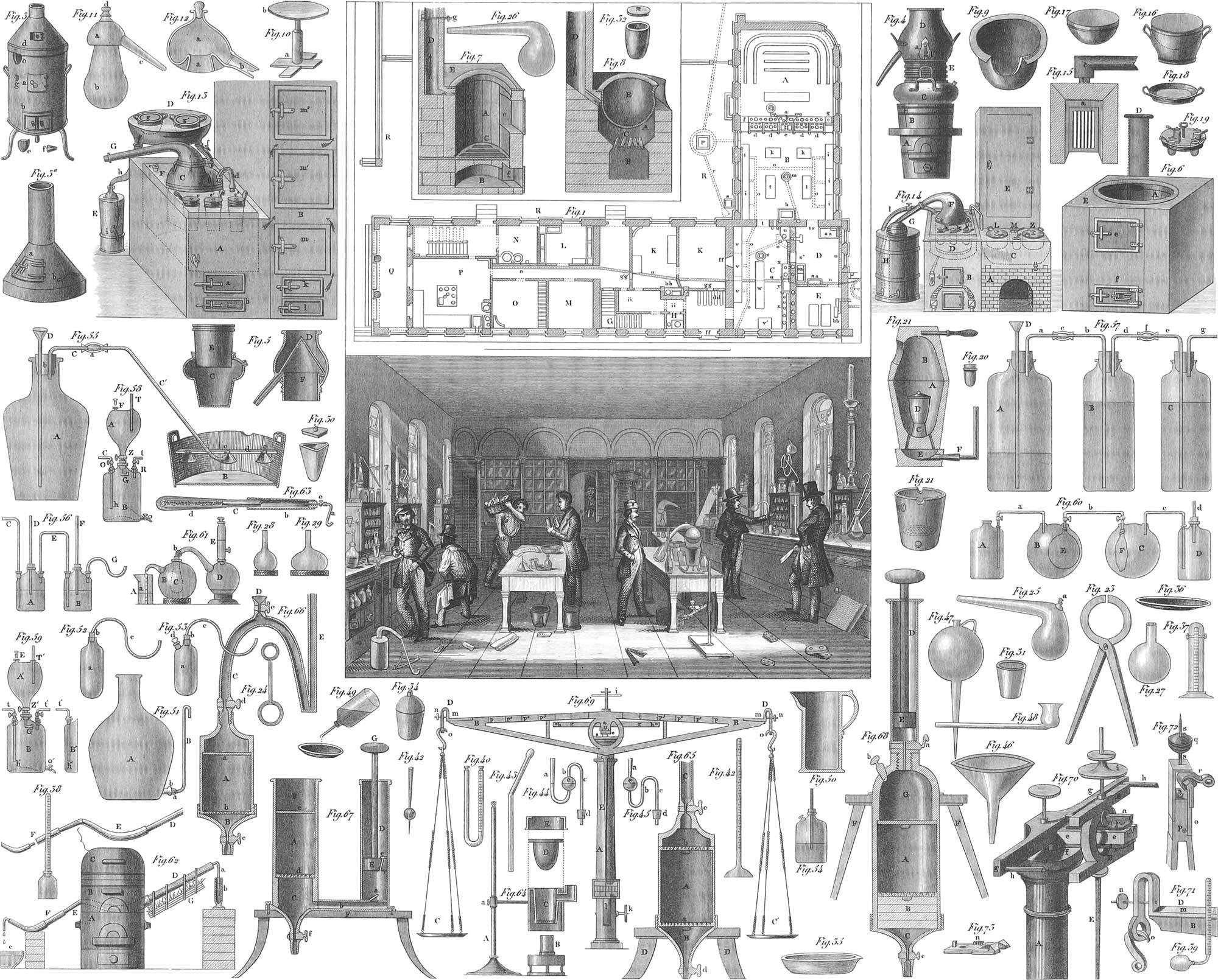
Contrivances for melting solid bodies have the most extensive application in the arts. All workers in metal need them for producing the various forms or mixtures required. The daily operations of life involve them to a very great extent. Confining our attention to what is required for the more infusible substances, among which the metals are most conspicuous, we have, as most generally used, the wind furnace (pl. 30, figs. 3 and 7), and the crucible furnace (figs. 21 and 22). Thus, when a mixture of copper and zinc is required in making brass, pieces of both metals are introduced into a Hessian crucible (figs. 30–34), constructed of an exceedingly infusible clay. Charcoal powder is introduced into the crucible to prevent the loss of zinc, which arises from its ready combination with oxygen. The philosophy of the action of the carbon consists in its taking hold of the oxygen of the air entering the crucible, and being converted by it into carbonic acid. The zinc is thus protected from the influence of the oxygen, which would have been exhibited by the conversion of the metal into an oxyde by combustion; combustion or burning, in technical phrase, simply indicating a combination of oxygen with any inflammable base. The crucible is now set on the grate of the portable wind furnace (fig. 3), placed a few inches above the door a, and surrounded by red hot coals. The space above is then to be filled with fresh coal, and the door b, leading to the space below the grate, opened; the door a must be kept closed, its object being to allow an examination of the interior of the furnace with respect to the temperature, amount of fuel, &c. The funnel or cap, represented more fully in fig. 3a, is finally to be laid on the upper opening of the furnace As soon as the fuel has commenced to burn, a powerful draught of air draws through the furnace, owing to the heated air within being specifically lighter than that outside, and ascending continually through the cap, its place being supplied by fresh air entering at b. A great quantity of atmospheric air, consisting of seventy-nine parts of nitrogen and twenty-one of oxygen in the hundred, by volume, is thus introduced among the burning coals. The oxygen combining with the carbon with great avidity, produces an increase of heat, and the carbonic acid being immediately carried up and out through the funnel, permits a fresh accession of oxygen. The amount of heat generated will be in direct proportion to the amount of oxygen which has access to the carbon of the fuel, and to the rapidity with which the carbonic acid is removed.
When many operations of this kind are required it is customary, besides or instead of the portable furnace, to use one that is fixed, but similar in principle and action. A furnace of this character is shown in pl. 30, fig. 6; fig. 7 represents it in section. A is the space for the fuel, C the grate on which stands the crucible, supported by a block of crucible earth, as in fig. 21; B is the bottom of the ash-hole. The opening of A is closed by a well-fitting iron cover, which may be coated on the under side with clay. The draught in this furnace passes in at the ash-pit, through the fuel, and out by a horizontal channel into the vertical chimney, D, which may be walled in the chimney of the building. By applying very high chimneys, a much greater draught can be obtained than with the preceding construction.
The crucible furnace (pl. 30, fig. 21) is still another arrangement, especially applicable when valuable metals, as gold and silver, are to be melted. The larger upper figure represents a small closed space, whose walls are formed of an infusible clay, and made in two pieces, the upper of which can be lifted off like a cover. The metal (or metals) to be melted is introduced into a crucible of plumbago, or into a Hessian crucible, and this placed on a block of infusible earth in the bottom of the furnace: burning coals are now to be placed round the crucible, and after replacing the top, the remaining space is filled with fresh coal. In the bottom of the furnace are six or eight channels, so arranged that the air forced by a pair of bellows into the space E, and thence through the channels, may be directed principally against the lower part of the crucible. The cover has a lateral aperture through which examinations may be made or fresh coal supplied. The crucible is removed after complete fusion of the metal has taken place. Fig. 23 represents a pair of tongs for handling the heated crucible. The lower figure, marked fig. 21, is a smaller crucible furnace for melting minute quantities of matter; its application follows readily from what has just been said.
In melting operations which are exclusively scientific, and not technical in their object, as, for instance, in ascertaining whether a substance can be melted by some of the fluxes at our command, we may in many cases make use of the blowpipe (pl. 31, figs. 62, 63). This consists of a brass tube inserted into one end of an expansion, a. into whose side is attached a smaller tube, b, with its extremity tapering to a very fine aperture. Air is blown from the mouth into the tube, and the extremity held above the wick of an alcohol lamp, so that the flame is driven into a horizontal pointed cone by the fine current of air: a very intense heat will thus be produced, and especially just before the point of the blowpipe. The substance to be tested is placed in small portions, in a hollow excavated in a piece of charcoal or clay, or held in platinum forceps, and the flame directed upon it. The object of the central chamber of the blowpipe, as just described, is to condense the moisture of the breath (the air too is condensed to a certain amount); there are sometimes several jet pieces, of different calibres, for slipping on b. Quite frequently the blowpipe consists merely of a tapering tube of brass, bent at right angles near the extremity.
The oxy-ethereal lamp (pl. 31, figs. 1 and 2) is capable of furnishing a heat equal in intensity to almost any with which we are acquainted. A is a glass lamp filled with ether; B a tube by which the air is brought into connexion with the inside of the lamp: C a fine metal tube, leading from a gasometer of oxygen, into the middle of the wick of the lamp. The lamp rests on a foot, D, through which the tube C passes. The wick of the lamp is covered by the ground-glass cap a, to prevent the evaporation of the ether when the apparatus is not in use. The heat of the lamp is capable of melting flint sufficiently to permit its being drawn out into a thread.
Other methods are employed when the heat required is not so intense as that we have just supposed. In many cases glass vessels well annealed may be used to much advantage.
Apparatus for Converting Solid Bodies into Gaseous
For the above-mentioned purpose, much the same apparatus may be employed as is used in vaporizing liquids, namely, alembics, matrasses, and retorts. An operation of the kind may, for instance, have for its object the separation of bodies from each other. Thus, if it be desired to obtain the valuable mercury used in the construction of mirrors from its amalgam, the latter must be introduced into an iron retort (pl. 30, figs. 25 and 26). The first form differs from the second in having a small tube, a, opening into the head of the retort, for the purpose of more conveniently filling it, especially in cases where the neck must not be soiled by the material introduced. After filling the tube is to be closed by an iron stopper or other appropriate substance. When the bulb of the retort is introduced into a furnace and heated, the mercury passes over through the neck into a receiver, cooled by a stream of water, where it is again condensed. The tin remains in the retort, and both metals may thus be obtained separately. An apparatus of iron, provided with necks of leaden or glass tubes, may also be employed.
Similar retorts, of glass, porcelain, or clay, may be used when the heat required for vaporization is not so great. Should it simply be required to drive off, without collecting, the more vaporizable of two substances, we may frequently make use of the matrass, constructed of iron, porcelain, glass, or clay. The bottom of such a vessel, into which the mixture has been introduced, is heated, and the vapor allowed to escape through the open neck, the heat being so managed as always to be below what would vaporize the second substance.
Operations of this kind are frequently instituted by the chemist, and often find application in the arts. Thus zinc, in this way, may be driven off from its combination with many other metals, as it evaporates at a comparatively low temperature. Sulphur, also, is expelled in a similar manner, although the heat required is much less than in the case of zinc. An operation of this kind is called a distillation when the vapor thus produced becomes converted into a liquid on cooling, and a sublimation when this vapor assumes a solid state, apparently without previously passing through the liquid. Distillation takes place much more frequently in the case of liquids than of solids.
Apparatus for Converting Liquid Substances into Gaseous
Nature exhibits to us on a large scale the conversion of liquid substances into gaseous. The evaporation of water is an instance of this kind. The most varied applications are made in Chemistry and the arts of that property of liquids by which ebullition and consequent evaporation take place at certain temperatures. The ebullition or boiling of liquids consists in the fact that, after they are heated to a certain point, any additional increment, instead of being sensible to the thermometer, is expended in a combination with individual particles of the liquid. Thus water, when heated, gives indication to the thermometer of a rise of temperature up to 100° C, 80° R., or 212° F. Arrived at this point, the temperature remains fixed, provided that no change takes place in the height of the barometer, and the additional heat, instead of elevating the temperature, appears to combine with the atoms of water, expanding them to such a degree as to convert them into vapor or steam. The heat disappearing in this manner is said 1o become latent, as it cannot be indicated by the thermometer, although actually existing. For when this vapor or steam is led into cold water, its consequent condensation is accompanied by a rise in the temperature of the water corresponding to the amount of heat rendered latent. The boiling point of liquids, or the limit of the ascent of temperature at which ebullition takes place, depends greatly on the pressure of the atmosphere, boiling taking place sooner as the pressure is less. Thus, on the top of Mont Blanc, water boils at 187° F.
The process of distillation is generally employed to separate liquids of different boiling points from each other. Thus, in the fabrication of whiskey, after the fermentation of the mash, a mixture is produced from which the alcohol formed is to be separated, and obtained tolerably free from water. Pure anhydrous alcohol boils at 172.4° F., water at 212° F. On bringing the mash into a distilling vessel, and regulating the fire so that the temperature of the vessel shall not rise to the boiling point of water, the latter cannot pass over in any great quantity as vapor, while the spirit evaporates as soon as its own proper temperature has been exceeded. By cooling the tube terminating the distillatory apparatus, the vapor will be again condensed, and a liquid obtained which contains a much larger proportion of alcohol than before. The spirit will not be perfectly pure, inasmuch as some watery vapor will pass over; the amount of this will, however, be less as the temperature at which the operation is performed is lower.
It is very evident that we may separate vaporizable liquids from substances mixed with them, provided the latter require a higher temperature for vaporization than the former. The usual apparatus of distillation on a small scale is the retort; for larger operations, the alembic or the still. For most distillations of liquids we may use retorts of glass (pl. 30, figs. 25 and 26). In fig. 26 the liquid is introduced by means of a tube which is longer than the neck of the retort; in fig. 25 by means of a funnel through the head a in the bulb of the retort, this aperture being closed by a well ground stopper. The retort thus arranged is placed in the sand bath furnace (fig. 8). The space E forms a sand bath composed of tin: it is exhibited more fully in fig. 9. On its bottom is placed a thin bed of dry sand, and the retort, after being set on this, is surrounded by an additional quantity of sand. The bath has a notch cut out of the edge to receive the neck of the retort. The vessel or tube applied to the extremity of the neck of the retort must be luted or cemented as closely as possible. The globes or matrasses (figs. 27, 28, and 29) are used for the purposes of receivers. In order that the neck of the retort may fit as accurately as possible into that of the globe, one of the latter is selected whose neck in one place is only a little wider than that of the retort to be set in it, and the neck of the globe broken off at this place with the help of a splitting iron (fig. 24). Of these irons there must be a considerable number at hand; to use them, one is selected fitting the neck of the globe, or indeed any other cylinder of glass, at a given place. It is then to be heated red hot and brought to the place in question, being held there for a few seconds. On removing the ring and pouring cold water on the heated glass, this will crack off evenly at the part which had been surrounded by the red hot iron ring. Having cut off the neck of the receiver to the proper length (it may possibly not require the operation at all), the neck of the retort is inserted into it, and the joining well luted. To support the receiver at the proper height, we may use a small table, as represented in pl. 30, fig. 10. The stem of this can move up and down in the cylindrical part, a, of the stand, and may be fixed at any height by a screw. The retort thus placed in the sand bath, with the receiver supported by the table, and generally resting on a ring of some kind for greater steadiness, heat is to be applied under the furnace and the receiver kept constantly cooled. The vapor arising from the ebullition, and passing over into the receiver, is there condensed again into a liquid.
For distillation on a larger scale we make use of the alembic. For this purpose the alembic is constructed in two different shapes. A very simple arrangement, and one long in use, is the small alembic represented in pl. 30, figs. 11 and 12. The vessel, b, in fig. 11 is intended to contain the liquid to be distilled. This is closed above by a head, a, shown more fully in fig. 12, well fitted to the body, and the junction luted to prevent the escape of vapor. On heating the bottom of the body, b, the vapor of the boiling liquid ascends into the head, and passes through the neck into the receiver, which is kept constantly cool. By means of an aperture in the head, closed by a glass stopper, fresh material may be introduced into the body without interrupting the operation.
A convenient arrangement for a distilling apparatus, which may be used for preparing distilled water, is shown in figs. 4 and 5. AB is the furnace over which the alembic, C, is heated. The part E fits exactly, with its under portion somewhat conical, into the opening of the alembic C (figs. 4 and 5); the head itself D (fig. 5), has the following arrangement: The interior of the cylinder E, which is in connexion with the space of the alembic and receives the vapor, is closed like a roof above, as seen in fig. 5. At the base of this roof is the escape-pipe. The space D is filled with cold water. On bringing the water in the alembic, C, to boil, the steam ascends to the roof over F, and is here condensed by contact, with the constantly cold walls, escaping by the pipe in the liquid form. In this arrangement the head itself forms the cooling apparatus.
A complete distilling apparatus, with a very convenient arrangement for cooling the vapors, is shown in pl. 31, fig. 3. A is an alembic, generally constructed of copper; B the head, which, with the tube C and the cooling vessel EE, may more economically be constructed of tin. The space for the fire is at G. As soon as a liquid boils in the alembic A, its vapors ascend into the head B, and thence through the tube C into the cooling apparatus. The latter consists of a wooden box, DD, in which is set a tin cylinder, EE, running out below into a tube., F. Into the cylinder, EE, is inserted a smaller tin cylinder, eeee, closed below, down whose middle passes the tube of a funnel, a, reaching almost to the bottom. An uninterrupted stream of cold water is allowed to flow into the under cylinder through the funnel, a, and its tube. The vapors from the alembic, which enter the space inclosed between the walls of the inner and outer cylinder, are cooled, on the one side, by the fresh water introduced through the funnel a, and, on the other, by the water in the box DD. Fresh cold water is introduced into the bottom of this box by means of the funnel, b, and the pipe, c. As the water in the inner cylinder, eeee, becomes heated, it flows through a small pipe into DD, and thence, with the heated water of DD itself, out at a. It will be remembered that water, as it becomes heated, becomes specifically lighter, for which reason the warmest water will always occupy the highest position in the vessel containing it. The cold water, also, introduced through the funnels, occupies the lowest part of the several vessels, and displaces the heated water previously occupying that same position. The vapors from the body of the apparatus are condensed so perfectly by this arrangement, that a liquid, hardly lukewarm, flows out as the result through the pipe F.
All distilling apparatus, properly so called, have for their end the recovery of the liquid vaporized. It often happens, however, that our only object is to collect some solid dissolved in a liquid. Thus, when we wish to obtain solid salt from a solution of salt and water, the water is here of no use. In such cases, instead of distilling vessels, we make use of very shallow and wide vessels, in which the liquid may come as much as possible in contact with the air. Evaporating dishes of this kind are represented in pl. 30, figs. 35 and 36.
Instruments for Investigating Matter Which is Gaseous at Ordinary Temperatures
We have already gone into some detail upon the methods of converting solids into liquids, and liquids into the gaseous condition. It remains to see whether the reverse may not be possible, whether it does not lie in our power to convert a gas into a solid, or at least into a liquid. With the means commanded by modern chemistry, there are now only three elementary substances which cannot be changed from their gaseous condition: oxygen, hydrogen, and nitrogen. The method of condensing gases will readily suggest itself from what has been said on the subject of boiling. Since the cause of the gaseous condition of a solid or liquid is to be found in the combination of heat rendered latent, and since, when this latent heat is withdrawn, the vapor again becomes a solid or liquid, we are fairly entitled to suppose that those substances which are gaseous at ordinary temperatures, owe this property to their latent heat; and we may legitimately presume that by removing this latent heat the conversion desired may be effected. The means at our command for removing latent heat consist in the application of an extraordinary pressure, since, in proportion to the amount of compression exerted, latent heat is rendered sensible, and thus by pressure alone may many gases be condensed into liquids. A second method is afforded by the use of the so-called freezing mixtures. By mixing together certain substances, an extraordinary amount of cold may be produced, and many gases rendered liquid by such artificial congelation. In cases which are very difficult to reduce, both methods may be applied simultaneously. Thus the gas may be placed in a cylinder of metal, in which fits a piston, air-tight, and depressed with great force at the same time that the cylinder is immersed in a freezing mixture. The condensation of carbonic acid gas may serve as an illustration of this method, as also of quite a peculiar mode of producing a great degree of cold. Carbonic acid gas is well known, by its effects at least, to everybody; it is one of the substances formed by the combustion of coal, and one which is developed in the fermentation of liquids containing sugar, as beer and wine, its presence being indicated in the frothing and foaming of these and similar liquids, when the cork inclosing them is withdrawn. Carbonic acid, to become liquid at the temperature of freezing water, or 32° F., requires a pressure of sixty atmospheres, and at a temperature of 86° one of seventy-three atmospheres. The apparatus employed is represented by pl. 31, fig. 6. A is a cylinder of cast iron into which bi-carbonate of soda must be introduced before the piece C is inserted. At the same time a copper vessel is to be introduced into the cylinder containing as much sulphuric acid as will suffice to expel all the carbonic acid gas from its combination with the soda, by combining itself with the latter. The iron piece C, having a longitudinal canal, is now crewed into the cylinder. The copper tube, mm, is so inserted into the side of C as to communicate with its canal. In a similar manner this tube opens by its other extremity into the piece D of the smaller iron cylinder B, similar in construction to C, and having its inner canal in connexion with the inside of the cylinder. The iron pieces, c, d, are inserted into the similar pieces C and D, and through them pass the screws, a, b, by means of which an attached valve fig. 7 (upside down in the plate), may be so adjusted, that the openings of the tube, mm, may be shut or opened at pleasure. The clamps n, n, and E, E, hold the two vessels tightly together. The apparatus being thus arranged, the opening of the tube, mm, in C is closed by depressing the valve by means of the screw a. The apparatus is to be inclined until the sulphuric acid placed in the copper vessel may flow over the bi-carbonate of soda, and thus produce a chemical decomposition. Both valves are now opened, or that connected with the screws a and b, and the carbonic acid generated passes into the cylinder, B, cooled by ice. So vast an amount of gaseous carbonic acid is generated from the bi-carbonate of soda, that this becomes fluid from the pressure produced by its own abundance. As soon as the action of the acid on the soda is supposed to be completed, the opening of B is closed by means of the valve at b, and the apparatus taken apart. On opening the valve at b, the liquified carbonic acid will flow out as soon as the cylinder B is inverted. The walls of the vessel must be strong enough to resist a pressure of sixty atmospheres, and the entire experiment is at all times dangerous. In an experiment of the kind, instituted in Paris by Thilorier, the apparatus burst and killed one person, and severely wounded several others. The liquified carbonic acid evaporates so rapidly when the pressure is removed, that a great part of it becomes solid, the part converted into gas rendering so much of the heat latent as to freeze the rest. In the condensation of carbonic acid gas, we have, therefore, an example of a gas condensed to a liquid by pressure, and, at the same time, the condensation of a liquid by cold into a solid body, the acid being actually frozen by the rapid abstraction of the heat required by the evaporation of the gas. Thilorier obtained solid carbonic acid in a white mass, similar to a ball of snow. By combining solid carbonic acid and ether, the acid becomes at first liquid, and then evaporates along with the ether; a cold of such intensity is thus produced as causes the thermometer to sink to 180° below the freezing point of water. Faraday has obtained a still lower temperature of 175° below the zero of Fahrenheit, or 207° below the freezing point of water, by the action of solidified carbonic acid in a vacuum. By the use of carbonic acid, Faraday succeeded in liquifying many other gases which until then had resisted every effort. Oxygen, hydrogen, and nitrogen, however, resisted every attempt of the kind, even when combined with enormous pressure. Liquified nitrous oxyde boiled on the application of the carbonic acid.
Many gases may be liquified on a small scale in bent tubes (pl. 30, fig. 43), at one of whose extremities a strong glass bulb is blown. Supposing the bi-carbonate of soda to be introduced into the bulb, and the sulphuric acid kept in the angle of the bend until the open end of the tube is melted together; on bringing the two in contact a rapid evolution of gas ensues, which results as before. One end, of course, must be placed in a freezing mixture.
Elementary Condition of Matter, or the Elements
Only a small amount of the matter surrounding us is in its elementary condition. Of the gaseous elements, two, oxygen and nitrogen, mixed in proportions of twenty-one to seventy-nine, form atmospheric air. Of liquid elementary substances, mercury is the only one which occurs in nature. On the other hand, many of the solid elements occur uncombined, as gold, silver, iron, platinum, &c. All these substances, however, occur more frequently in a compound state, or united together, and by far the greater number of these chemical combinations consist of one element, combined with one or more others in a definite proportion. The material composing the substance of plants and animals almost always consists of suck combinations. Hence we see that to ascertain the properties of an elementary body, we must carefully separate it from its combinations. In the following articles we shall present the principal methods of doing this for a number of these elements.
Apparatus for Obtaining the Gaseous Elements
Oxygen gas, while in a state of mixture, and not of combination, is a principal constituent of atmospheric air and the great instrument of organic vitality. Inhaled into the lungs, or brought into contact with the respiratory apparatus of animals in general, whether skin, gills, lungs, or branchiæ, it furnishes the chief means for purifying the blood by eliminating the effete carbon. This gas, however, does not exist alone. The stimulus to these various organs would, in many cases, be too great in the case of pure oxygen alone: we therefore find this gas diluted with a large proportion of nitrogen.
So intimate, however, is the mixture of oxygen and nitrogen in the air, that it is impossible to separate them by mechanical means; it therefore becomes necessary to do this by chemical agencies, which may also be applied in obtaining oxygen from other mixtures or compounds, the latter, in fact, being by far the best sources from which to procure the gas in question. Oxygen combines with almost all the other elementary bodies; at least, the only one in respect to which this fact has not been ascertained, is fluorine. To obtain oxygen pure, therefore, we may use one of several different combinations. Thus, for instance, we may select the protoxyde of mercury, a reddish substance, known in the arts as red precipitate. A sufficient quantity of this is introduced into the retort, b (pl. 31, fig. 13), the neck of this being firmly held by the clamp of the stand C. The neck of the retort terminates in the balloon, c. in which it is secured by a cork. From the second opening of this balloon a bent tube, d, passes into the water of the trough A. On one of the shelves of the trough is placed the receiver B, first filled with water, and then inverted so as to keep it entirely full; the end of the tube, d, must be just under the bottom of the receiver B. The inversion of the receiver, when filled with water, will be more practicable if the open end be closed by a plate of ground glass, which is to be removed under the surface of the water in the trough. After seeing that all the joints are rendered perfectly air-tight by means of the proper luting, a red heat is applied to the bottom of the retort by means of the spirit lamp a. At the temperature of a low red heat, the red oxyde of mercury is decomposed into its elements, and the gaseous oxygen first drives out the contained air through the conducting tube, d; for this reason the first bubbles, when formed, must be allowed to escape and not pass into the receiver. When all the air has been expelled, the succeeding oxygen passes from the end of the tube d, and rising through the water in a succession of bubbles, occupies the top of the receiver, displacing as much water as the space it occupies. When the receiver, B, is filled, it is to be removed to another shelf, and replaced by a new receiver, prepared like the last.
The philosophy of the operation by which the oxygen is liberated is as follows: Oxygen has a very great tendency to maintain the gaseous condition, and we have seen that the ordinary agents of cold and pressure, as usually applied, have been unable to effect its condensation into a liquid. In this case chemical affinity has done what the other agents failed to accomplish, namely, changed it from a gas into a solid. The accompanying mercury, also, is in a solid state, instead of its ordinary liquid condition. Heat, however, overcomes this combination by which the two elements are solidified, and the elements, expanding, resume their more natural state. The mercury is liquified; the oxygen converted into gas, which then passes over. The heat applied must of course not be. sufficient to vaporize the mercury: this requires a temperature of 660°F.
A more economical way of procuring oxygen is to employ black oxyde or deutoxyde of manganese in the apparatus given in pl. 31, fig. 60. The iron retort, C, is filled with manganese, and placed in the furnace, B. In its mouth is inserted the bent iron gun-barrel or leaden tube, leading to the pneumatic trough, D. On heating the retort to redness, one portion of oxygen is liberated from its combination with the manganese, and driven over to the trough, where it is to be collected with the precautions described in the preceding paragraph.
Hydrogen is likewise a gaseous elementary body. While oxygen occurs abundantly in the atmosphere combined mechanically, and in the solid and liquid constituents of the earth combined chemically, hydrogen is never met with but in the latter state of combination. We may indeed make a slight exception for the minute quantity discernible at times in the atmosphere, the result probably of volcanic action. It is most abundant in a combination with oxygen forming water. The proportions of these two gases, in water, are one part of hydrogen and eight of oxygen by weight, and two of hydrogen and one of oxygen by volume. The readiest way of obtaining hydrogen consists in the decomposition of water. Pieces of zinc are introduced into the gas generator, A (pl. 30, fig. 55), the vessel filled about two thirds full of water, and sulphuric acid poured in by degrees through the funnel tube, D. The water standing a little higher than the lower end of the funnel tube D, closes this tube, so that the gas, when generated, can only escape through the tube, CC′, which is inserted into the cork stopper of the vessel A. In the case of this generating vessel, and, indeed, in all apparatus used for developing gases, some arrangement is employed to prevent any danger of bursting by the stoppage of the conducting tube. This purpose is accomplished in our illustration by means of the funnel D, out of which the liquid will be forced by any accumulation of gas in A. Another contrivance, called Walter’s Safety Tube (figs. 44 and 45), is intended for the same purpose. Water, or any more appropriate liquid, is poured into the leg a (fig. 45), so as to fill the bulb except about one third. A safety tube of this character is inserted into the corked stopper of the generating apparatus instead of the funnel D (fig. 55). Should any obstruction occur in the conducting tube, the gas escapes by the safety tube. The column of liquid which under satisfactory circumstances stands about e, and in the tube at b, is then driven back into the bulb. The gas then ascends through the liquid in the bulb in the form of bubbles, and thus escapes. The arrangement of fig. 44 may serve to bring about the same result in regard to the conducting tube, by attaching to the latter an apparatus similar to that of pl. 31, fig. 61 (the figure is inverted in the plate). The end, a, of the latter apparatus is to be placed in the cork of the generating vessel. Pl. 31, fig. 56, exhibits still another kind of gas generator, with two openings, one of them receiving the funnel tube, the other the gas conductor. It frequently happens that gases must be freed from the watery vapor with which they are combined. This is done by interrupting the gas conductor by a wider tube (fig. 56), first partly filled with cotton, and next with pieces of chloride of calcium, the latter substance absorbing watery vapor with great eagerness. The tub B, in pl. 30, fig. 55, is the pneumatic tub or trough as generally used by chemists. It has at cc a shelf, provided with grooves and holes, under some of which small funnels are attached. Water is poured into the trough to a height of an inch or two above the shelf. The receivers are to be filled with water, and then inverted and placed on the shelf, over one of the apertures. The end of the tube communicating with the gas-generating apparatus is to be brought under one of the funnels, which then guides the gas in its ascent through the water to the top of the receiver. The general operation is the same as already described. The receiver may also be so placed on the shelf as to project by less than half its diameter, and the end of the gas tube brought under the open space. In this way the holes and funnels may be dispensed with.
Chlorine, at ordinary temperatures, is a gas of a yellowish green color, and very corrosive, and poisonous when inhaled. This gas is one of the constituents of common salt, which is a combination of chlorine with the metal sodium, forming, in chemical nomenclature, the chloride of sodium. Chlorine is very conveniently obtained from muriatic or hydrochloric acid, an acid procured in large quantities in the manufacture of carbonate of soda from common salt. This hydrochloric acid is a combination of chlorine and hydrogen, formed under the conditions required for separating the chlorine from common salt. Fill the flask A (pl. 31, fig. 14) nearly half full of hydrochloric acid, and into it drop some substance rich in oxygen, as the peroxyde of manganese: the oxygen of the latter will combine with the hydrogen of the acid, forming water, leaving the chlorine free to pass over, and to be collected in a receiver, as already described. To accelerate the operation, a spirit lamp is placed under the flask, which is fixed by the two rings, a and b, of the retort stand, C. The apparatus (fig. 14) here described is applicable to many other purposes.
Apparatus for Procuring the Liquid Elements
Among the simple substances entering into the composition of our earth, and its contents, only two are liquid at ordinary temperatures; the non-metallic bromine, and the metallic mercury. The latter is sometimes found native, but more frequently in its combination with sulphur as cinnabar. The following method may be employed to separate the metal from the sulphur. Mix the cinnabar with iron filings, and place it in an iron retort (pl. 30, fig. 25). The retort is then to be brought to a red heat, as in a wind furnace (fig. 3). At this red heat the sulphur combines with the iron filings, forming a sulphuret of iron, leaving the mercury in a metallic condition to be carried over in the form of vapor into the neck of the retort, and thence to an appropriate receiver. The receiver being kept constantly cool, the vapor of the mercury is condensed, and the metal thus obtained in its ordinary liquid state.
Apparatus for Obtaining the Solid Elements
The methods to be employed for separating those elementary bodies which are solid at ordinary temperatures, vary very much with the different combinations which have to be considered. Only a few of these methods of manipulation can be referred to in the following pages. Pl. 31, fig. 8, represents the apparatus for obtaining phosphorus. BC is a furnace, into which an iron retort, A, may be introduced. The retort must be filled with a mixture of charcoal dust and phosphate of lime. This latter salt is obtained from burnt bones, by several intermediate stages of manipulation. The phosphoric acid at a red heat is decomposed, and the oxygen having then a greater affinity for the carbon of the charcoal, forms with it carbonic acid and carbonic oxyde, leaving the phosphorus in the form of a vapor, which is carried over and condensed in the bent upper tube, b. The end of the tube dips a few inches below the surface, ccc, of the water, placed in a flask, D, closed above with a cork, through which passes the tube d. The phosphorus thus condensed in the tube flows into the water, where it accumulates at the bottom. Great care is necessary to prevent any access of atmospheric air, as the contact of the two would be followed by a combustion resulting from the combination of the phosphorus with oxygen, and the consequent formation of phosphoric acid. The tube d, which, passing through the cork, does not reach quite to the surface of the water, serves to permit the escape of the various gases, as carbonic oxyde and acid, which are formed during the operation. Phosphorus is a non-metallic substance having much the appearance of white wax, and requires to be handled very carefully on account of its inflammable character.
Preparation of Sodium. Common salt, rock salt, sea salt, all one and the same substance, consist of two simple bodies combined in definite proportion, the one a gas, chlorine, the other a silver white metal, sodium. The latter has so great an affinity for oxygen as to take it from almost any combination possible to be formed. Thus, if sodium be thrown on water, a part of the latter will immediately be decomposed, its oxygen uniting with the metal to form the alkali soda, and the hydrogen escaping in the form of a gas. The combination, however, of sodium with water, is attended by the development of so much heat, as to inflame the liberated hydrogen, which immediately unites with the oxygen of the atmosphere. In the fabrication of hydrochloric acid from common salt, the base of the latter is finally obtained in the form of an oxyde, or as the alkali soda, known as caustic soda. This is, however, not obtained in its separate form without further manipulation, being exhibited as a carbonate. It is from this carbonate of soda that the metal may be obtained by means of the furnace DD (pl. 31, fig. 9). The wrought iron vessel, A, is to be laid on the furnace on the two cross-bars, f, f. One of the iron bottles used to contain the mercury of commerce may be used as the vessel in question. Into the opening of this bottle, a gun-barrel, about six inches long, must be firmly fixed, and the bottle partially filled with a mixture of dry carbonate of soda and charcoal, and placed in the furnace, which must have a very powerful draught. The opening, CC, through which the bottle is introduced into the furnace, is closed tight by a piece of fire-brick, any interstices being luted or cemented. The short iron gun-barrel, a, passes through the brick. When the vessel has been brought to a red heat, the copper vessel, B, is joined to the gun-barrel, a, by a short neck in the upper portion, and partly covered by a wire frame. Opposite to the neck just referred to, is a short copper cylinder at e, extending to the wire frame, the object of which is to permit the introduction of an iron rod through B into the tube a, for the purpose of freeing it from any obstruction. The tube, d, is attached to the side of B, near its upper part. This copper vessel, B, consists of two portions which can be lifted apart. The lower part embraces the upper as far as the roof, cc, which is soldered nearly in the middle of the upper part of the vessel. The lower part of the vessel is now to be filled about two thirds with petroleum or naphtha, the upper part slipped in, and the vessel thus adjusted, brought into communication with the iron tube a. The wire frame must be kept constantly cold by means of snow or ice, and the heat of the furnace raised to a great intensity. At a strong red heat the carbonate of soda is decomposed into carbonic acid and oxyde of sodium, and the carbon of the charcoal combines with the oxygen of the oxyde, forming carbonic oxyde and carbonic acid, leaving the metal free. The gases pass over through the tube into the cold copper vessel; the metal in the form of vapor also passes over, and is condensed into small globules, which fall to the bottom of the naphtha. The naphtha being a hydrogen compound, free from oxygen, prevents the access of the latter gas to the metallic sodium. A nearly similar process may be used to obtain some other metals, as potassium, from their combinations.
Method of obtaining Metallic Iron. Our object in this place is not to treat of the reduction of iron on the large scale from its ores, but simply to mention the chemical process by which we are enabled to ascertain the amount of pure metal in a certain combination, such as the protoxyde or peroxyde.
For this experiment we may use the apparatus represented in pl. 31, fig. 58. A glass tube, b, constructed of glass of difficult fusion, and having a bulb blown in the middle, has small brass cocks attached to each end by caoutchouc tubes. By means of these cocks the tube is to be attached to an air-pump, and afterwards weighed when all the air has been removed. Note must be taken of the loss of weight in the tube, produced by the exhaustion of the air. Into the bulb of the tube is now to be introduced a quantity of still hot oxyde of iron, heated over a Berzelius lamp. This lamp (fig. 11), one of the most convenient of all chemical apparatus, is constructed as follows: a is an annular vessel for containing alcohol, the space within the annulus being occupied by a cylinder, c, in communication with the vessel. The cylinder is double, one within the other: an annular bottom is soldered between the two cylinders, thus inclosing a space which is brought into communication with the space of the vessel a, by means of two short tubes passing between a and c. A tubular lamp-wick is placed in this space between the two cylinders, and kept constantly moistened by the alcohol flowing from a through the tubes; this wick may be regulated, as to height, by a screw, f. The space within the inner cylinder is open at both ends, thus allowing the introduction of a constant stream of fresh air into the centre of the wick when burning. The flame is surrounded by the small chimney g, made of sheet iron, and intended to increase the draught. The lower slide, d, of the stand, e, carries the lamp, and another above it the vessel to be heated, in this instance the crucible b. Both slides may be set at any height along the vertical rod of the stand, by means of lightning-screws. The iron pincers (fig. 64) are used to handle the heated vessel.
If now, as already mentioned, a sufficient quantity of oxyde of iron, as from thirty to fifty grains, be removed from the crucible into the tube b (fig. 58), the oxyde of iron is to be permitted to cool in a vessel filled with dry air; the cocks are to be again attached, and the air pumped out, after which the tube is to be again weighed. The excess of weight in the latter weighing will represent the weight of the oxyde introduced. After again removing the cocks the glass tube is to be connected by dried cork or caoutchouc tubes, with the rest of the apparatus shown in fig. 58. Here A is a gasometer filled with hydrogen; B a flask half full of sulphuric acid; a a bulb tube containing chloride of calcium; then comes the tube with the oxyde of iron, and to this succeeds the tube c, likewise filled with chloride of calcium. After this series of tubes has been connected air-tight by means of the glass conducting tubes, f, f, f, the cock, e, of the gasometer is to be opened. The hydrogen passes out in bubbles through the sulphuric acid into the top of B, thence through f into the first chloride of calcium tube a. The sulphuric acid through which the gas is driven abstracts from it the mingled watery vapor; this, however, is done more completely by the chloride of calcium, the gas arriving perfectly dry in the tube b, where it comes into contact with the oxyde of iron. As soon as all air in the apparatus has thus been replaced by hydrogen, the lamp (fig. 11) is to be brought under the bulb of b, and the oxyde of iron heated to a slight glow. When this has ensued the hydrogen combines with the oxygen of the oxyde to form water, which is carried by the succeeding hydrogen into the calcium tube c, and there absorbed. After a time the oxyde in b will be found completely reduced to metallic iron. The lamp must now be removed and the whole apparatus allowed to cool. The bulb containing the iron is again to be provided with the cocks and the air exhausted; on weighing it, the difference between this weighing and the preceding will be the weight of the oxygen, and the difference between this last weighing and the first will be the weight of the iron. In experiments tending to great accuracy, the chloride of calcium tube, c, is likewise weighed before and after the operation. This tube will be increased in weight by the water absorbed, and as the composition of water is well known, the amount of oxygen in the estimated weight of water must coincide with the amount lost by the oxyde of iron.
The Elements and Their Combinations
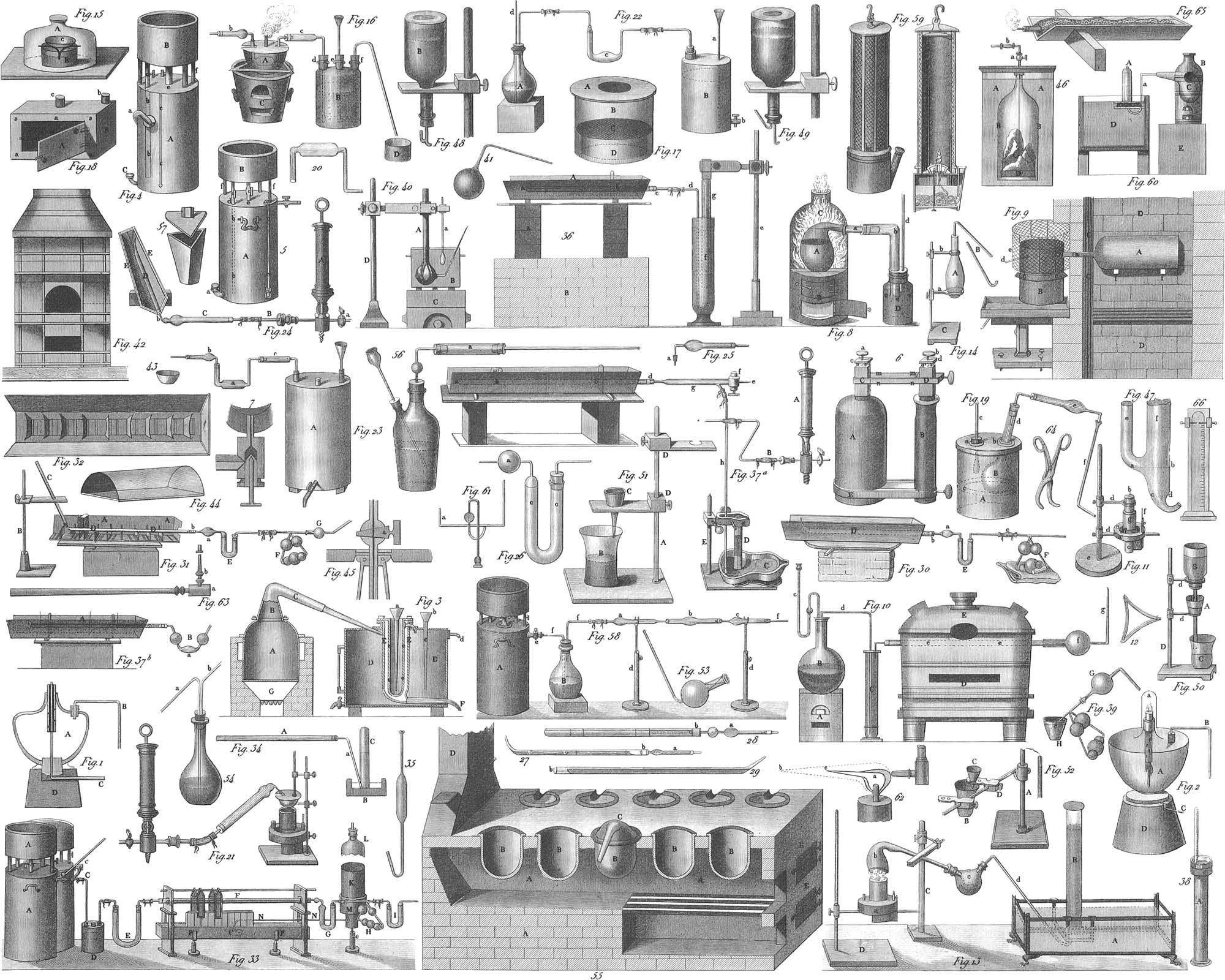
It has already been remarked that by far the greater number of bodies surrounding us are chemically compound in their character. Of this we are abundantly convinced by the possibility of reproducing certain compounds from the elements which we had obtained from them. In this way we are enabled very conveniently to form certain substances whose composition has been first ascertained by analysis.
It is a very general, if not universal law, that a simple body combines only with another simple body, rarely with one that is already compound. In this manner are produced chemical combinations of the first, degree, or binary compounds, containing two elements. When two binary compounds unite, a ternary compound is produced. The number of these ternary compounds far exceeds that of any of the others.
Different names are given to particular classes of these compounds. Thus the binary compounds of oxygen with any other element are either oxydes or acids. When the same element combines with oxygen to form an oxyde in more than one proportion, that containing the least quantity of oxygen is called the protoxyde; the next, deutoxyde; the third, tritoxyde; &c.: the highest proportion gives us the peroxyde. The acid combinations of oxygen have the name of the combining element with a termination of ic. Thus nitrogen and oxygen form nitric acid. If there be two acid compounds, the one with least oxygen ends in ous, as nitrous acid.
The most remarkable law of chemistry, and at the same time the one on which the whole science depends, is. that the elements always combine in definite proportions by weight. An entirely new attribute is thus added to our previous idea of an element, namely, its capacity of combining, according to definite laws, with all the others. Thus carbon never combines with oxygen in any other ratio than that of 6:8, while sulphur combines with oxygen in the proportion of 16:8. The same numbers, six and sixteen, expressing the ratio of combination for carbon and sulphur with oxygen, express also the ratio of their mutual combination. Their ratios experience no other modifications than those obtained by multiplying the first or second term. This same law of definite proportions applies not only to carbon, sulphur, and oxygen, but to all elements. Knowing, then, the ratio in which all the elements combine with oxygen, we shall have the ratio of their combinations with each other. This ratio may be expressed either by the numbers themselves, or by their multiplication into some definite progression. This progression may be the series of numbers from one to five, and in some cases fractions intermediate to these. The numbers thus obtained for the different elements are known by the terms, atomic weights, combining numbers, or chemical equivalents, and were first established with remarkable accuracy by Berzelius, and afterwards corrected by other chemists. In the following table we present the elements already known to chemists, with their symbols and equivalents. For greater convenience we have given two series of equivalents, the one where hydrogen is taken as unity and oxygen as 8, the other assuming 12.5 as the equivalent of hydrogen, and 100 as that of oxygen.
The equivalents adopted in the table are taken from the third volume of the fifth (German) edition of Berzelius’ Treatise on Chemistry, a few more recent determinations by Marignac being added. Many chemists consider the equivalents of Calcium, Magnesium, Iron, Silver, Mercury, Sulphur, and some others, as exact multiples of that of Hydrogen; but experiment has not yet established this point in a perfectly satisfactory manner.
Tantalum, Niobium, and Pelopium occur associated in nature, and have not yet been perfectly separated. The name Columbium was applied to the metal obtained from the Columbite of Connecticut by Dr. Wollaston. Rose has recently ascertained its identity with Niobium, and it is therefore better to drop the old name, which has only been employed by American writers.
The symbols are abbreviations of the English or classical names, employed for greater convenience of reference. Those elements to which no equivalent is appended, have either been discovered quite recently, or else elements supposed to be pure, have been ascertained to be compounded with some other elements, and the equivalents previously determined must therefore be rejected.
The circumstances under which chemical combinations of simple bodies take place, are very various. Temperature exerts a great influence, although its action differs much under different circumstances. Many bodies require to be cooled in order that a combination may take place. Thus chlorine only combines with water at and under a temperature of about 32° F.; others combine directly at ordinary temperatures, whenever brought into communication, as potassium and oxygen. By far the greater number of combinations, however, are produced through the instrumentality of heat, while others again are decomposed by the same agency. Of all simple elements, oxygen exhibits the greatest tendency to combine with the rest, and it is combinations of oxygen which constitute the greater portion of the mineral, vegetable, and animal kingdoms. Many compounds of oxygen are produced at elevated temperatures alone. Under these circumstances, the heat which is liberated in almost all chemical combinations, and especially those between elements having a great affinity for each other, is set free in large quantities, thus serving to heat fresh matter. Many substances do not combine quite so readily with each other, and with many the temperature must be elevated to a very great degree to bring about the desired result.
Names | Symbols | H = 1 | O = 100 |
---|---|---|---|
Aluminum | Al. | 13,67 | 170,9 |
Antimony | SB.(1) | 129,03 | 1612,9 |
Arsenic | As. | 75,10 | 938,8 |
Barium | Ba. | 68,54 | 856,7 |
Bismuth | Bi. | 212,85 | 2660,7 |
Boron | B. | 10,89 | 136,2 |
Bromine | Br. | 79,96 | 999,6 |
Cadmium | Cd. | 55,73 | 696,7 |
Calcium | Ca. | 20,12 | 251,6 |
Carbon | C. | 6, | 75,0 |
Cerium | Ce. | 47,26 | 590,8 |
Chlorine | Dl. | 35,45 | 443,2 |
Chromium | Cr. | 26,30 | 328,8 |
Cobalt | Co. | 29,48 | 368,6 |
Copper | Cu.(2) | 31,64 | 395,6 |
Didymium | D. | 49,60 | 620,0 |
Erbium | E. | ||
Fluorine | F. | 19,0 | 237,5 |
Glucinum | G. | 13,93 | 174,2 |
Gold | Au.(3) | 98,16 | 1229,1 |
Hydrogen | H. | 1 | 12,5 |
Iodine | I. | 126,88 | 1586,0 |
Iridium | Ir. | 98,56 | 1232,0 |
Iron | Fe.(4) | 28,04 | 350,5 |
Lantanum | La. | 47,04 | 588,0 |
Lead | Pb.(5) | 103,56 | 1294,6 |
Lithum | L. | 6,52 | 81,6 |
Magesium | Mg. | 12,64 | 158,1 |
Manganese | Mn. | 27,56 | 344,6 |
Mercury | Hy.(6) | 100,09 | 1251,2 |
Molybdenum | Mo. | 46,0 | 575,0 |
Nickel | Ni. | 29,54 | 369,3 |
Niobium | Nb. | ||
Nitrogen | N. | 14 | 175,0 |
Norium | No. | ||
Osmium | Os. | 99,40 | 1242,6 |
Oxygen | O. | 8 | 100 |
Palladium | Pd. | 53,23 | 665,4 |
Pelopium | Pe. | ||
Phosphorus | P. | 31,36 | 392,0 |
Platinum | Pt. | 98,56 | 1232,0 |
Potassium | K.(7) | 39,10 | 488,8 |
Rhodium | R. | 52,15 | 651,9 |
Ruthenium | Ru. | 52,15 | 651,9 |
Selenium | Se. | 39,61 | 495,2 |
Silicon | Si. | 22,21 | 277,7 |
Silver | Ag.(8) | 107,96 | 1349,6 |
Sodium | Na.(9) | 23,17 | 289,7 |
Strontium | Sr. | 43,67 | 545,9 |
Sulphur | S. | 16,05 | 200,7 |
Tellurium | Te. | 64,13 | 801,7 |
Tantalum | Ta. | ||
Terbium | Tb. | ||
Thorium | Th. | 59,50 | 743,8 |
Thin | Sn.(10) | 58,82 | 735,3 |
Titanium | Ti. | 24,12 | 301,5 |
Tungsten | W.(11) | 95,06 | 1188,3 |
Uranium | U. | 49,42 | 742,8 |
Vanadium | V. | 68,55 | 856,9 |
Yttrium | Y. | ||
Zinc | Zn. | 32,52 | 406,6 |
Zirconium | Zi. | 33,57 | 419,7 |
- Stibium
- Cuprum
- Aurum
- Ferrum
- Plumbum
- Hydrargyrum
- Kalium
- Argentum
- Natrium
- Stannum
- Wolfram
Apparatus for Combining Gaseous Elements
Combination of Hydrogen with Oxygen. To ascertain the ratio in which these two gases combine, we make use of an instrument termed an eudiometer (pl. 31, fig. 66). This is especially employed to determine the character of a gas with respect 10 its amount of oxygen. The eudiometer is essentially a graduated glass tube, into the upper end of which two wires have been inserted opposite to each other. A tube of this character is often surrounded, excepting two longitudinal strips allowing the graduation to be seen, by a metal casing. This tube is first inverted and filled with water, then erected under water and raised, so that the lower part of the tube may still be immersed, the tube itself remaining filled with water. Pure hydrogen gas is now to be introduced into the tube by the ordinary method of manipulation, filling it to a certain amount, as twelve degrees; if half this volume or six degrees of pure oxygen be now introduced, and the charge of a Leyden jar passed through the mixed gases by means of the two metal wires, an explosion will take place, sometimes sufficient to shatter the tube. If the gases be perfectly pure, the water will immediately rise to the top of the eudiometer, the contained gases having all been combined to form water. If seven degrees of oxygen had been taken in the above experiment, then there would have been one degree of oxygen left in the tube after the explosion, just as we would have had a portion left of hydrogen had this gas entered in greater proportion than the one mentioned. Here we perceive that chemical combinations are all definite, and that an excess of one ingredient over the proper proportion, is indicated by this excess remaining free.
Apparatus for Combining Liquid Elements with Gaseous
As already mentioned, mercury and bromine alone of all chemical elements, occur in the liquid condition. Mercury can be combined directly with gaseous oxygen. If this metal be boiled for several months in a matrass (pl. 30, fig. 27), whose neck for this purpose should be several feet long, the mercury will be gradually changed into a red crystalline powder, which, in 108 parts by weight, contains 100 of mercury and 8 of oxygen. It is this oxyde of mercury which by heating is again decomposed into mercury and oxygen, and may therefore be used in the preparation of pure oxygen. More advantageous processes for obtaining this oxyde are known in the arts, more complicated, however, in their character.
Apparatus for Combining Solid and Gaseous Elements
Among the gaseous elements, oxygen and chlorine, as also iodine, bromine, and sulphur, when converted into vapor by heat, combine with almost all other elements; at least in many cases all that is necessary to effect this combination is the heating of the latter. The apparatus employed for the purpose is generally very simple, consisting principally of crucibles, tubes of porcelain and glass, capsules, matrasses, flasks, and retorts.
In most experiments, however, instituted with the gases proper, a large quantity of these is required to be kept in convenient vessels. Such vessels are known as gasometers. They are so contrived as to permit a convenient filling in of the gas, as well as its safe preservation for a considerable length of time when not required for use. To this must be added a facility in extracting as much or as little as may be needed for experimental purposes. Pl. 30, figs. 58 and 59 represent a gasometer of the earlier construction. The entire apparatus, consisting of two vessels, is first filled with water, and the cock, Z, closed, so that the water in the flask cannot flow out through the tube G. The gas conductor is attached to the tube C, through which the gas generated is allowed to enter. The cocks O and o are opened, R remaining closed. As the gas enters through the tube CO into the vessel B, and rises in single bubbles through the water in this vessel, the water is driven out through the open cock, o, at the bottom. As soon as B is filled with gas, the cocks, O, o, are closed, and the apparatus retained in this state until the gas is required for use. To force out the gas when wanted, the cock Z must be opened. The air which presses on the flask through the tube T, after the openings E (fig. 59) and F (fig. 58) have been closed, drives the water through the bent tube, G, into the gas-vessel, B, here displacing the gas, and driving it out through Rt when the cock R is opened. The gas may thus be driven into another vessel, as shown in fig. 59, by connecting the extremities of the two brass tubes, t′, t′, by caoutchouc.
The gasometers of more recent construction are, however, far more convenient (pl. 31, figs. 4 and 5). The first figure gives a back, and the second a side view of the instrument: A and B are cylindrical vessels made of copper or zinc. The upper one is connected with the lower by posts and several tubes. The rods e and bb are tubes, provided with cocks, by means of which the communication through the tubes between the two vessels may be interrupted or restored at pleasure. One tube, bb, reaches nearly to the bottom of the vessel A; the other, e, ends on the cover to which it is soldered. The glass tube, cc, is so attached to the outside of the lower vessel, as that one end communicates with the interior of A at the top, and the other end at the bottom. At the bottom of A there is an escape pipe, C, capable of being closed by a screw lid. To use the apparatus, the cocks at e and b are first opened, and the apertures in (fig. 5) a and (fig. 4) C are closed by their cover. Water is poured into the upper cylinder, which enters through the tube bb into the vessel A, while the air ascends through the tube e, and escapes in the form of bubbles. When the entire apparatus is filled with water, all the cocks are closed, and the screw C opened. As the air has no access to the space in A, its pressure on the small surface at a or C keeps the water in equilibrium, so that this cannot escape. The tube from the gas-generating apparatus is introduced into C, and the gas allowed to ascend. Occupying the highest part of A, the gas displaces the water, driving it out through the aperture C (or a). The tube c serves to indicate the height of the water in the vessel A, and consequently the volume of gas. When the gasometer is filled, the opening C is closed. When the gas is required for use it escapes through a tube attached to the right of fig. 5. which is provided with a cock. On pouring water into B, and opening the cock d, the water will descend to the bottom of A and press upwards against the gas. This will then readily flow out on opening the cock of the lateral tube.
It has already been mentioned that oxygen and hydrogen unite in proportions of one measure of the former and two of the latter to form water, whenever the electric spark is passed through the mixture. A mixture in these proportions is known as the explosive gas. The union of the two gases takes place with an extraordinary development of heat, and in the moment in which watery vapor is formed by the combination so sudden an expansion is produced, that large quantities of gas sometimes involve serious explosions. The heat generated in this combination is the most intense which chemistry can produce; many substances formerly deemed infusibie, being readily melted when exposed to this flame. An arrangement by which the two gases can be burned together without danger is called an oxy-hydrogen blowpipe. A simple arrangement of this kind may be readily understood from what has been said on the subject of the gasometer. One gasometer must be filled with oxygen, another with hydrogen, and the two so arranged that the escape gas pipes from both vessels may stand at an equal height and a little inclined to each other. Then on opening the proper cocks in the two, a current of gas will escape from each, which being inflamed together or being allowed to pass through the flame of a spirit lamp, will produce a heat so great as to melt a wire of platinum. Several contrivances may be employed to prevent any danger of explosion which might arise from the inflammation of a mixture of the two gases. A direct mixture of the gases before combustion would be perfectly safe by causing them to pass through Hemming’s safety tube, a tube filled compactly and entirely with fine cylinders of wire, so that the gas must pass through the exceedingly fine tubes formed by the interspaces between the cylinders. Gurney’s apparatus of safety may also be used.
Apparatus for Combining Solid and Liquid Elements
As has already been remarked, there are only two elementary bodies which are liquid at ordinary temperatures; these are mercury and bromine. By far the greater number are solid. With respect to the latter we may say in general that one solid element does not combine with another. The condition of aggregation of bodies that are to combine together must be liquid or gaseous, a condition to which solids must be brought. Consequently we may consider the combinations of liquids and solids under one head, provided the solids be supposed to be rendered liquid by heat. Setting aside the gases, we have as non-metallic elements, boron, bromine, iodine, carbon, phosphorus, sulphur, selenium, and silicium; all the rest are metals. As far as any brief generalities can be made respecting the combinations of these simple substances, we may say that almost all the non-metallic elements combine in definite proportions with the metallic when brought together in a liquid state, while the non-metallic bodies, as well as the metals, either come together in any proportion, or else do not unite directly into a homogeneous whole. The instruments in which experiments of the kind are conducted, are retorts (pl. 30, fig. 25, and 26), matrasses (figs. 27, 28, 29), and crucibles (figs. 30, 32, and pl. 31, fig. 57), the application of all which has already been explained.
Chemical Synthesis and Analysis. The Apparatus Required.
It has already been remarked that simple bodies do not often combine with compound, but compound unite with compound just as simple with simple. In by far the most cases we have in chemistry to deal with the combinations of compound bodies, and in chemical analysis we obtain two binary compounds as the result, as for instance a combination of oxygen and a metal on the one hand, and of oxygen and a non-metallic body on the other. The compounds of oxygen with the non-metallic bodies, sulphur, carbon, nitrogen, are generally acids; the combinations of oxygen with metals are rarely acids, and exhibit in most cases a great tendency to unite with acids to form a salt. Potash, for instance, consists of carbonic acid and oxyde of potassium or pure potassa; the former being composed of carbon and oxygen, the latter of oxygen and potassium. This potassa has so great a tendency to combine with carbonic acid as to seize hold of it whenever the two come into contact, to form the carbonate of potassa. These oxydes which stand in such a relation to acids are called bases, the compound itself is a salt. The tendency of one base to combine with two or more different acids is very different, this difference being indicated by the terms greater or less chemical affinity. It is hence readily intelligible that an acid which has a greater affinity for one base than another, may take the former from a combination which it may already have formed. On this principle depends the development of carbonic acid gas in the generating apparatus (pl. 31, fig. 46), which at the same time constitutes a very simple gasometer. In the glass cylinder A is placed a second cylinder, B, whose superior opening is fastened to the cover of AA, and, connected with a pipe provided with a cock, may be closed or opened by means of a cock at a, seen also in fig. 45. The wire C is suspended from a small hook in the tube, and carried beneath the bottom D, which is thus suspended a little above the lower end of B. By placing pieces of carbonate of lime (limestone, marble, or chalk) in the bottom D, and filling the cylinder A about half full of dilute sulphuric acid, the liquid will rise in A as soon as this is set in B. On opening the cock a, the dilute acid will rise still higher in B, driving out the inclosed air and covering the carbonate of lime. As sulphuric acid has a greater affinity for lime (oxyde of calcium) than is possessed by carbonic acid, the latter will be displaced by the former, and will be liberated in the form of carbonic acid gas, the sulphuric acid combining with the lime to form a sulphate of lime. The evolution of the carbonic acid gas will depress the liquid in B, causing it to rise in A, and finally this depression will be so great as to leave the lime uncovered by the dilute acid. Further evolution of gas will then stop until the cock a is opened for the escape of carbonic acid gas, this escape being facilitated by the pressure of the liquid in A. As the gas escapes, the liquid is again enabled to attack the carbonate of lime, and to generate fresh gas, as before. This apparatus thus serves an excellent purpose in enabling us to keep constantly on hand a small quantity of this and some other gases, as hydrogen, for immediate use.
An apparatus by means of which nitric acid may be manufactured on a large scale is represented in pl. 31, fig. 55. It consists principally of a furnace of peculiar construction, called a galley furnace, the general relation of whose parts is shown hj the figure. AA is the masonry inclosing the inner furnace space. D the chimney, F and E the fuel and ash-doors. BB indicates a series of cups in which glass retorts, as CB, are set. The retorts are filled half full of nitrate of potassa or saltpetre, and sulphuric acid poured in through a tube reaching nearly to the bottom of the retort. All the cups being provided with retorts properly prepared, and these provided with properly cooled receivers, heat is applied to the whole row by means of a single fire. As soon as the mixture in the retorts is raised to a certain heat, the sulphuric acid takes the potassa from the nitric acid, forming sulphate of potassa, and leaving the nitric acid free to escape into the receivers, there to be condensed.
An illustration of an entirely different method of separating one substance from another is seen in the ordinary assaying of silver and gold. If, for example, it be desired to ascertain how great an amount of silver is contained in any object, as a coin, some grains of the object are placed with a certain quantity of lead in a cupel (fig. 43) composed of bone earth and ashes. In mints and silver mines, where such operations occur daily, several such cupels are placed in a clay muffle (fig. 44). This muffle is then set in the opening, A, of the muffle furnace (fig. 42), and surrounded by red hot coals. In the glowing current of air within the muffle, the lead and copper (if copper compose the alloy) are oxydizeu, one portion of the oxyde of lead escaping in vapor, the rest, however, melting with the copper, and being absorbed by the cupel. The silver remains pure in the form of a round granule, which is weighed, and the amount of weight compared with that ascertained before the operation.
Having thus mentioned the principal generalities, with respect to the combinations of simple and compound bodies, we shall pass to the consideration of some more complex chemical operations. Thus, supposing it be required to obtain the metal aluminum from clay, which is its oxyde, we shall not be able to procure this directly from the clay itself. Nevertheless, we may replace the oxygen of the alumina by chlorine, and then we may abstract the chlorine by some metal, as potassium, which has for chlorine a greater affinity than is exhibited by aluminum: this metal will thus be left separate, and resembling iron in general appearance. First of all, then, to replace the oxygen of the alumina by chlorine. For this, as well as many other purposes, we employ the apparatus represented in pl. 31, fig. 10, where A indicates a small furnace on which the matrass B is heated. In this is placed a mixture of powdered oxyde of manganese and hydrochloric acid, which, when heated, will generate chlorine for some hours: c is a safety-tube to prevent the bursting of the matrass by any obstruction in the tube ee. The chlorine developed passes through the glass tube, d, into the cylinder C, half filled with water, in which it deposits the impurities brought from B, and thus purified goes into the porcelain tube, ee. Into this tube have previously been introduced small cylindrical pieces of alumina, prepared by mixing with a solution of sugar or paste, and baking in a covered crucible until the sugar or paste is completely carbonized. The furnace, D, which, after being filled with coal, and fired up, is covered with its top, E, is intended to bring the alumina in the tube to a red heat. At this temperature the former affinities are suspended, and the oxygen of the alumina combines with the carbon of the carbonized sugar, forming carbonic acid; the aluminum being left in a metallic condition, is immediately seized by the chlorine. The chloride of the aluminum being liquid at a red heat, flows into the cooled receiver, f, attached to the end of the porcelain tube, ee; the gaseous carbonic acid likewise escapes through the tube g.
Chemical Examination of Organized Bodies, and the Apparatus Necessary for this Purpose
The material forming the bodies of plants and animals is known as organized or organic, in contradistinction to the inorganic matter found in the mineral kingdom. Excepting the hard parts of the animal, as the bones, teeth, &c,. and the ashes of the plant, which latter form a very slight percentage of its mass, the principal part of the animal body consists of but four elements: nitrogen, oxygen, carbon, and hydrogen; and of the vegetable, but three: oxygen, carbon, and hydrogen. The method of manipulation by means of which we may ascertain the amount of the individual elements in an animal or vegetable body, will be briefly described, and may serve as an example of the complicated and laborious investigations necessary for the purpose. Let the problem be, carefully to ascertain the proportions in which the four elements enter into a portion of animal matter, or the three into a part of a plant. The first requisite in solving this problem is a very accurate weighing of the amount of the substance to be subjected to analysis. All substances, however, in contact with the air, absorb a certain amount of watery vapor through their pores, which necessarily requires a careful drying of the body as an absolutely necessary preliminary to this weighing, else we should be in error by the weight of the water contained, which would be dissipated in the course of the operation. This removal of water is effected by means of the following forms of drying apparatus:
The Drying Apparatus with Sulphuric Acid pl. 31, fig. 15
This consists of the receiver A. fitting air-tight on a plate like that of the air-pump, and of the capsule, B, placed beneath the receiver, and half filled with concentrated sulphuric acid. The object of the acid is to remove moisture from the inclosed air by reason of its great affinity for it. The substance to be dried is placed finely powdered in a porcelain capsule, upon a triangle of iron wire (fig. 12) resting on the dish containing the acid, and is then to be left for several days. The moisture of the body gradually exhales in the perfectly dry air, and the capsule loses in weight by the amount of the water exhaled and absorbed by the sulphuric acid. Fig. 17 shows a still more advantageous construction of this apparatus. B is a large glass vessel with a ground edge; D, the contained dish with the sulphuric acid; C, a wire frame upon which the capsule with the object to be dried is set; A is a ground-metal cover, closing air-tight on the edge of B, previously rubbed with tallow. In its centre is an aperture, likewise capable of being closed by a ground-cover, and through which small objects may be introduced into the apparatus. For such substances, however, as sugar, which are capable of experiencing a pretty high heat without decomposition, other apparatus of quicker operation may be employed. Such are the water-bath, the oil-bath, and the air-bath (pl. 31, figs. 16, 18, 19, 20, 21). First, the water-bath (fig. 16). A is a copper vessel, with a properly fitting cover, possessing two intersections to receive the two legs of the glass vessel (fig. 20) set in A, and containing the substance to be dried. To one leg is connected the chloride of calcium tube c, which is continued into a tube bent at right angles; to the other leg is likewise attached the chloride of calcium tube b. The former is fitted air-tight by means of cement in the cork of one opening of the Wolff apparatus B. The second opening, e, of the bottle contains a funnel tube, and in the third, f, is fixed a syphon. The kettle, A, is to be filled with water, so that the vessel (fig. 20) containing the substance to be dried may be completely covered, and the water brought to boil by means of the furnace C. At the same time a vessel filled with water is placed above the funnel-tube, e, from which water may be made continually to drop into the funnel. The water thus entering soon covers the bottom of the syphon tube, and drives the air from the bottle, B, into the chloride of calcium tube c. in which all the vapor contained in the air in B is retained. A stream of perfectly dry air then passes through the vessel containing the substance in question, and soon takes up all the moisture which may be there. The chloride of calcium tube b absorbs the water thus abstracted from the substance. By extracting the cork containing the funnel-tube, and starting a current of water from the syphon, the water in B may be removed, and the operation of furnishing a current of dry air again continued as before.
Other apparatus is at the command of the chemist for producing a current of perfectly dry air for such purposes. Fig. 22 shows at A a flask partly filled with sulphuric acid, connected by means of conducting tubes with the drying vessel and the aspirator B. The latter is a cylinder of sheet-copper or zinc, provided below with a cock, b, and above with a funnel-tube, a. This is filled entirely with water through the funnel-tube a, and the funnel then closed by means of a cork. On opening the cock b, the water will flow out of the cylinder B, since the air entering through the tube d ascends through the concentrated sulphuric acid into the vessel A, and is thus dried; it afterwards passes over the substance placed in c, to fill the space left vacant in the aspirator B by the withdrawal of the water.
The apparatus (pl. 31, fig. 23) is used in a similar manner, differing only in that the vessel of sulphuric acid is replaced by a chloride of calcium tube, b, in which the inhaled air is dried. Finally, in fig. 21, the aspirator is replaced by an air-pump. The body is placed in a small matrass, immersed in the kettle. From this there leads a chloride of calcium tube for drying the air left by the air-pump after extracting the watery vapor. In other particulars, the operation of this apparatus is similar to that already described. By means of these forms of apparatus we are enabled to dry a substance at the boiling point of water; other contrivances are necessary, however, when the heat required, and possible, is greater than 212° F. Thus sugar at 212° indeed loses all the water mechanically combined, but at a temperature of 329° F., it loses a definite proportion of chemically combined water, and the determination of this is of great importance with reference to the nature of this substance. For the purpose of exposing this and other bodies to a higher temperature, then, than that of boiling water, we make use of the oil bath (fig. 18), or the air bath (fig. 19.)
The oil bath (fig. 18) consists of a quadrangular box with double walls, about an inch apart. The intervals, a, a, a, a, between these walls, are filled with oil introduced through the aperture, c. In the opening, b, is set a thermometer, with its bulb dipping into the oil, so as to enable us to ascertain the temperature; the bottom of the box is heated by the furnace on which it is to be set. The substance placed in a capsule, is introduced into the space within the box, and the door A shut. By means of the thermometer, the heat may be so regulated, as not to exceed a certain temperature. Place, for example, a weighed vessel of sugar within this apparatus, and weigh it from time to time; we shall find that it decreases in weight for a while, and that after a time this weight remains stationary, the loss being not an arbitrary amount, but exactly proportioned to a given quantity of sugar.
The air bath (fig. 19), intended to answer the same purpose as the last, is still more convenient. A, is a cylinder of sheet copper, within which a ring, c, c, is laid. The upper part of this cylinder is closed by a copper cover provided with two openings. In one opening, a, is set a thermometer, and through the other the neck of a glass matrass of known weight is passed, its bulb supported by the ring, c, c. Into this matrass the substance is introduced, which is to be dried at any temperature. The opening of the matrass must be closed by a cork through which passes a fine glass tube to be connected with a tube, e, filled with chloride of calcium. The cylinder, A, is heated by a subjacent lamp, and a definite temperature thereby communicated to the air surrounding the matrass in the cylinder. The conducting tube, f, is brought into communication with an air-pump, and while the matrass with its contents is heated in the air bath, the air-pump from time to time extracts the moisture-charged air. The air which would return into the matrass on opening the air-pump, must first pass through the chloride of calcium, and will be there dried before it can enter into B.
By means of the apparatus just described, we are enabled to separate the water from any body whether chemically or mechanically combined with it. All the water being thus removed from the body, suppose the problem to be, to determine its remaining components. If sugar be the substance in question, take a certain amount and dry it in one of the ways just described. Pure oxyde of copper is next to be well heated in a crucible (pl. 31, fig. 57), and the known weight of sugar mixed with it, after the covered crucible has been allowed to cool to 250–260° F. This mixture of oxyde of copper and sugar is to be introduced into a tube (fig. 29) from one half to two feet long, made of the most infusible glass, the lower end of which is drawn out into a fine closed point. As both the oxyde of copper and the sugar absorb fresh moisture during the mixture, this must first of all be again removed. This is done by the application of the air-pump (fig. 24). This pump, A, is made to communicate by a tube, B, with the chloride of calcium tube (fig. 25), this tube itself inserted air-tight by the help of a pierced cork, a (fig. 25), into the mouth of the tube (fig. 29), after it has been filled with the mixture of oxyde of copper and sugar. The tube, as shown in fig. 24, is placed in a box, E, E, and surrounded with sand previously heated to 250°. The heat of the sand is communicated to the tube, D, which contains the mixture, and the water previously absorbed becomes vaporized. By means of the air-pump, A, the air contained in D, and charged with vapor, is carried through the chloride of calcium tube, C, in which the vapor is retained. On opening the cock, a, of the air-pump, the air again enters the tube, D, perfectly dried by the chloride of calcium, and is charged afresh with moisture. In this way the mixture of the two substances in D becomes after a time perfectly dry. The tube, D, or the combustion tube, is now separated from the chloride of calcium tube, C, and laid in the combustion furnace (fig. 30). This is a box of sheet iron, about two to three feet long, and one half foot broad at the upper and open top, being somewhat narrower below. The bottom of this is seen in fig. 32, to be pierced transversely to permit the air to come in contact with the burning coals; there are also notched transverse partitions, upon the edges of which the combustion tube is to rest, as seen in fig. 31; in this figure the anterior wall of the furnace, A A, is supposed to be removed. In the opening, b, of the combustion tube, DD, is inserted by means of a dry cork, a chloride of calcium tube, a (pl. 31, fig. 26), filled to cc, or else the simple chloride of calcium tube (figs. 27 and 28), as also figs. 30, 31. To this tube again is fixed the so called potash apparatus, F (fig. 30). This consists of a glass tube in which five bulbs are blown and arranged, as shown in the figure. The three bulbs lying in a row are filled with solution of potassa by dipping one end, c (fig. 39), into a vessel, H, containing solution of potassa, and sucking up the ley by means of the sucking tube, G, attached to the other end. When the potash apparatus is filled with ley, it is attached to the chloride of calcium tube, E (fig. 30), by a caoutchouc tube, c. Finally the combustion tube, D, is gradually surrounded entirely with burning coals, applied first anteriorly and then with great caution along the tube. The entire arrangement has the following end in view: The oxyde of copper with which the sugar has been mixed, gives off, as soon as it has been heated to redness in the combustion tube, its oxygen to the carbon and hydrogen of the sugar, forming with the former, carbonic acid, and with the latter, water. The oxygen naturally contained in the sugar likewise combines with the two other elements. The oxygen and hydrogen then of the sugar, together with the oxygen of the copper, all enter into combination, and are entirely converted into watery vapor and carbonic acid. Both of these substances converted into gas by the heat, pass over into the chloride of calcium tube, E. A great portion of the watery vapor readily condenses by communication with the air, and collects in the bulb a; the remainder is entirely abstracted by the chloride of calcium filling the apparatus to c, c, fig. 26. The carbonic acid enters into the potash apparatus, in which it is entirely absorbed. The combustion tube, D, and the chloride of calcium tube, E, still contain some carbonic acid which must also be brought into the potash apparatus. For this purpose the fine point of the combustion tube is broken off, and over the aperture is placed a glass tube, C (fig. 31), open at both ends, and sustained by a holder, B. The suction tube, G, or fig. 53, is applied to the potash apparatus, and a current of air drawn through the tube, C, into the combustion tube, DD, which gradually draws over into the potash apparatus the whole of the carbonic acid, there to be absorbed. The chloride of calcium tube, E, had been weighed before the operation; it is now again to be weighed, when the excess of weight will express the amount of water which was formed from the hydrogen of the sugar in its combustion with the oxyde of copper. The potash apparatus has likely been previously weighed, and its excess after the experiment will be the amount of carbonic acid formed from the carbon of sugar. The combining numbers of both water and carbonic acid are already known; in nine parts by weight, water contains one part of hydrogen and eight of oxygen. The amount of water ascertained, divided by nine, will give the amount of hydrogen contained in the given weight of sugar. Carbonic acid consists of three parts by weight of carbon and eight of oxygen; multiplying the amount of carbonic acid as ascertained, by the fraction
A special mode of treatment is required in the case of certain liquid and at the same time volatile organic matters, as alcohol, which cannot be mixed with oxyde of copper without loss. These are inclosed in little glass bulbs drawn out into a long tail, and one or two of these bulbs filled with the substance in question are laid in the combustion tube, as shown in pl. 31, fig. 27. The bulbs are covered with oxyde of copper, the tails being broken off. The rest of the operation is as in the case of dry organic matter. To the question which is answered by the analysis of a volatile organic body, there is almost always united another with respect to the specific gravity of its vapor, so that the determination of this always accompanies the analysis. For this purpose we make use of a balloon (fig. 41) provided with a long tube bent nearly at right angles. After weighing this w r ith the inclosed air we pour in enough of the liquid, the density of whose vapor is to be ascertained, for the volume of the vapor generated to exceed the volume of the balloon. This is then fastened by wire to the post, A (fig. 40), carried by the holder, D, and the balloon immersed in the oil bath, B, whose temperature is observed by means of the thermometer, a. The oil bath is heated by the furnace, C, to above the boiling point of the liquid in question, and at the moment when all the liquid in the balloon has been converted into vapor, the point of the tube is melted together by a blowpipe. The balloon is then allowed to cool and again weighed. Its present weight is that of the balloon and the contained vapor, which has driven out. all the air; the former weight was that of the balloon and the contained air. The volume of the balloon is determined by filling with distilled water and weighing. We can readily ascertain the volume occupied by a known weight of distilled water, and consequently the volume of our balloon, and hence also the weight of the included air of the empty balloon. Thus we. get, the weight of the vapor which occupies the same space as the air of the balloon.
There are numerous substances which do not completely burn with oxyde of copper alone. For such we can make use of a current of oxygen which may be passed over the oxyde of copper during the combustion. As the whole process and its accompaniments are much as we have already described, we shall here only mention the apparatus especially necessary. In pl. 31, fig. 33, AA represents a gasometer filled with oxygen gas. Next to it is a gasometer filled with air, communicating with the tube, C, through a tube, c, so that by opening the proper cocks, either oxygen or air may be allowed to pass through the apparatus. The tube, C, carries the gas into the bottle, D, containing sulphuric acid, and from this into the potash apparatus; both these vessels, with their contents, are intended to free the oxygen and the air from the watery vapor which may have been dissolved in them. The combustion tube, F, which contains the substance to be burned, mixed with oxyde of copper as before, is heated by means of a brass spirit lamp, PP. Enough spirit is poured into the cup, M, to fill the lamp, PP, to a proper height, the communication between the two being through the tube, NN. A glass bottle is inverted over the cup, M, so as to permit a glass tube passing through the stopper, L, to dip somewhat into the spirit in M. As soon as the level of the spirit in the cup sinks, that in the bottle flows out, and in this way its level in the lamp, PP, remains the same. The substance is burned as formerly, and the only difference between this and the preceding method consists in the passage of a current of oxygen over the oxyde of copper. In this is involved the apparatus, I, placed next to the potash apparatus. It consists of an U-shaped tube filled with pieces of potassa. This, as well as the chloride of calcium tube, and the potash apparatus, is weighed, and its object is to take up a certain amount of water carried off by the dry oxygen in passing through the potash apparatus. Furthermore, since all this apparatus had been weighed before the experiment when partly filled with air, and since the space occupied by the air is partly filled by oxygen (one tenth heavier than air) after the combustion, this gas must be again replaced by air for the final weighing. This is done by means of the second gasometer, after the cock of the first has been closed.
Substances which, like most that belong to the animal kingdom, contain nitrogen in addition to the rest, require this new element to be determined. After its amount of carbon has been ascertained, a second mixture of the substance with oxyde of copper is brought into a combustion tube (pl. 31. fig. 34). Only a part of the substance introduced into the combustion tube is burned at first, the resulting gaseous products being used to expel the air occupying the tube. As soon as this has been driven out, the remainder of the substance is burned, and the gaseous mixture furnished by the body together with the oxyde of copper, is collected. This is done by means of the tube, a, which carries the mixture into a graduated cylinder, C, filled with mercury. The cylinder being filled about one third with the gas, and the amount measured by the graduation on the tube, a sufficient quantity of solution of potash is taken up in the pipette (fig. 35), and thus introduced into the cylinder, C. The object of this is to remove the carbonic acid from the gaseous mixture, which consists of carbonic acid and nitrogen, after the condensation of the major portion of the watery vapor; in this way we are enabled to ascertain the relation of volume between these two gases. By calculation we can extend this ratio to the relative amount by weight of the two gases, and as the carbon has already been determined by a preceding analysis, we shall have the data necessary to determine the quantity of nitrogen corresponding to the carbon already found.
Another method of determining the nitrogen consists in directly burning a weighed amount of the substance to be investigated, and after procuring the entire amount of the contained nitrogen, to measure it. For this purpose we may use the apparatus shown in fig. 36. The combustion is conducted in the usual manner in the combustion tube ab, which is filled from a to h with a mixture of the substance with oxyde of copper, and from b to c with copper turnings. The solution of potassa is placed in the vessel f, which incloses the graduated tube g, also filled with the solution. As all the products of combustion of a substance composed of carbon, hydrogen, nitrogen, and oxygen, can only consist of water, carbonic acid, and nitrogen, there remains in the graduated tube g, after all the carbonic acid has been abstracted by the potassa, only the nitrogen, which may then be measured. In this experiment, it is true that the air has passed over from the combustion tube into the graduated tube g, but since the volume has been determined no error will arise from this mixture with the nitrogen. The tube a reaches with its bent leg nearly to the cover of the graduated cylinder g, and as soon as the combustion tube, ab, is cooled, as much of its gaseous contents passes from the cylinder g into the combustion tube as is necessary to fill it. The tube g being divided into cubic inches, or cubic centimetres, we can readily calculate the weight of the contained nitrogen, knowing the weight of a cubic inch or centimetre.
The volume of nitrogen may be more accurately determined than in the preceding method by means of the apparatus (pl. 31, fig. 37a). The combustion tube, a, b, c, d, is filled from its posterior extremity, a to b, with carbonate of lead, from b to c with the mixture of oxyde of copper and the substance to be burned, and from c to d with copper turnings. The first half of the carbonate of lead is heated, by which means its carbonic acid is liberated, and the air thereby expelled from the whole series of tubes. To assist in the expulsion of air from the tube, the air-pump A, with the tube B, is applied to the intermediate joint of brass, provided with the cock f, this joint carrying at one side the vertical glass tube h, a little over twenty-eight inches in length. The lower end of this tube dips into the mercurial trough C. The cock, f, is to be opened and the air pumped out from the whole apparatus, by which means the mercury ascends to a height of nearly twenty-eight inches in the tube h. The cock, f, is now to be closed, and the carbonate of lead heated red hot, until the carbonic acid liberated depresses the mercury in h again into the trough, and escapes from the mercury in bubbles. The cock, fi is then again opened, and the previous operations repeated, until all the air has been expelled by means of the carbonic acid gas and the air-pump. The cock is now to be closed, and the bent lower extremity of the tube, h, brought under the cylinder D, filled with mercury, this being held erect by the holder E; the substance also is to be burned by means of coals surrounding the combustion tube, a, b, c, d. As soon as a considerable quantity of gas, consisting of watery vapor, carbonic acid, and nitrogen, has accumulated, a sufficient amount of solution of potassa is introduced into the cylinder D by means of a pipette; this solution will take up both the water and the carbonic acid, leaving the nitrogen, which, however, will still contain a little vapor of water. Afterwards, to measure the amount of nitrogen, we must introduce a shallow dish under the opening of the cylinder, and remove it with the cylinder from the trough, the mouth of the cylinder still remaining closed by the mercury filling the dish. In this way the cylinder is brought into a high vessel (fig. 38), A, filled with water, and the dish removed. The mercury will fall to the bottom, and the gas remain included by the water. The graduated cylinder is depressed in the water until the surface of the water inside stands just at the same height as that in the vessel A; the number of cubic inches or centimetres contained is then to be read off.
All these methods for nitrogen depend upon measurements of gases, which can never be so accurate as weighing, as they are exposed to many more errors of observation, since, besides the direct volume occupied, we should have reference to the height of the barometer and thermometer. The following method furnishes the nitrogen in a form in which it may be weighed: let fig. 37b represent the combustion furnace with the contained combustion tube, A. Into this tube is introduced the substance to be burned, mixed, however, with carbonate of soda instead of oxyde of copper. On heating the mixture the organic matter is burned, at the expense of the water combined chemically with the soda, this water giving off its oxygen to the carbon of the organic body, and its hydrogen to its nitrogen. Ammonia results from this latter combination, which is received in the apparatus B, inserted in the combustion tube, A, by a cork, and filled with hydrochloric acid. A salt (chloride of ammonium or sal-ammoniac) is produced by the combination of the ammonia and hydrochloric acid, which is not volatile at the boiling point of hydrochloric acid, so that after pouring out the liquid from B into a capsule, the sal-ammoniac may be obtained separate by evaporation. This is then dissolved in dilute alcohol, and chloride of platinum added, which, combining with the chloride of ammonium, forms a double salt, the double chloride of platinum and ammonium, which is insoluble in alcohol. This double salt, after drying, is weighed, and from this weight the weight of the contained nitrogen may readily be determined.
Nitrogenous substances are generally of animal origin, and often contain sulphur and phosphorus in their composition. To; obtain these substances in a form in which they may be weighed, they must be converted into acids; for which purpose we may employ the apparatus, pl. 31, fig. 65. The substance whose proportion of phosphorus is to be ascertained, must be mixed with soda and saltpetre, and placed in a combustion tube, there to be burned. The carbonic acid formed during combustion escapes through the anterior open end of the tube, and in the reliquiae the sulphur will occur as sulphuric, and the phosphorus as phosphoric acid, which may then be separated.
Mechanical Separation, and the Apparatus Necessary
Use is often made in chemistry of methods which do not strictly belong to the operations of chemical analysis. Thus, suppose the oxyde of iron to have been precipitated from a solution of iron, and suppose it be necessary to weigh the former: the first condition necessary will be to separate the oxyde from the liquid from which it had been precipitated, and from the substances used in causing the precipitation. The liquid is first poured on a paper filter laid on the funnel, A (pl. 31, fig. 50), and allowed to run off into a tumbler, C, placed beneath, the precipitate remaining on the filter. The latter, however, contains a part of the liquid, as also of the substance causing the precipitation, both of which must be removed. This can, in most cases, only be done by long continued washing in distilled water. An arrangement is therefore needed which shall allow an uninterrupted current of pure water to flow on the precipitate, so as to wash entirely away from it anything soluble in water. For this purpose, we make use of the two-branched tube (pl. 31, fig. 47), which is inserted into the cork of a bottle filled with water, as seen in fig. 48, B. The tube (fig. 47) is immersed up to about ab in the liquid in the funnel, A (fig. 50). The two slides, d, d, can be fixed on the vertical rod, D, at any height, so that the washing bottle, B, may have any appropriate position. When the level of the liquid in the funnel A has sunk so far that the tube (fig. 47) is immersed only to cd, the column of water, abcd, higher by ac or bd, has a tendency to settle at a height equal to that of the liquid in the funnel A, the atmosphere pressing on the column through the tube e. It therefore flies out, and in the space thus reached there enters a bubble of air through the tube e. Now as air is much lighter than water, this bubble immediately ascends into the tube, f, filled with water, and enters into B (figs. 48, 49, and 50). By this the water in the funnel A again rises to the height ab, this action being continued uninterruptedly. When, however, the water level in the funnel is the same with that within the tube ab, the action of the tube (fig. 47) ceases spontaneously; for as soon as the column abcd no longer flows out, the remaining column in the tube f is supported by the pressure of the atmosphere acting through the tube e, like the mercury of a barometer. The adhesion of the water to the conical part of the tube likewise facilitates the action of the tube, so that the column abed stands generally a little higher than the surface of the water in the funnel A. This two-legged tube may easily be replaced by two tubes set close together in a cork, the action being the same in both arrangements. An arrangement of this kind is intelligible from an examination of fig. 49.
A simple filtering apparatus is shown by figs. 51 and 52. A represents the stand, B a tumbler into which the liquid is filtered, C the funnel, D differently arranged slides, which, by means of screws, can be fixed at any elevation on the vertical rod of the stand A.
In washing the precipitate, instead of the double-legged tube of the preceding apparatus, we may make use of the washing bottle, as shown in pl. 30, fig. 49, and pl. 31, fig. 54. In the first of these we blow into the bottle, half full of water, thereby compressing the contained air. On quickly inverting the bottle the pressure of the air forces out the water in a fine stream from the pointed glass tube which had been inserted into the aperture of the bottle by means of a cork. The water escapes, therefore, in a jet, and by directing this upon the filter the precipitate is quickly stirred up and well washed. The second apparatus (fig. 54) serves the same purpose, only the air is blown in through the tube b, and the water driven out through the tube a, which reaches nearly to the bottom of the bottle half full of water.
Funnels (pl. 30, fig. 46). The filtering funnels used in chemical operations are generally constructed of glass. Their broad portion is so fixed as that two opposite lines, drawn from the border to the apex of the cone portion, shall form nearly a right angle with each other. In the earlier chemical manipulations special funnels were employed, such as the one in pl. 30, fig. 48.
Separating Funnels (pl. 30, figs. 42 and 47) are instruments for separating two liquids which do not mix, and which possess different specific gravities, such as oil and water. The two liquids are poured through the upper opening into the vessel, and the latter closed air-tight by a well fitting stopper. The whole must be allowed to rest quietly until the liquids have separated into two layers. The lower aperture is then to be opened and the inferior layer permitted to escape. On closing the superior aperture with the finger the upper layer will remain in the vessel.
Florence Flasks (pl. 30, figs. 51 and 54). The essential idea of these flasks consists in such an arrangement that in a tube open above and below, or in a flask itself, liquids may continually flow to and fro without the level of the liquid in the tube or flask sensibly changing. Flasks of this kind are used to separate a small amount of a specifically lighter body from a great quantity of a specifically heavier one. Thus, let water distilled over plants containing volatile oils flow into the tube of the flask while the lower end of the tube remains closed by the water in the flask; then the oil will constantly remain floating within the tube, the water itself entering the flask until it flows out of a lateral opening. In a similar way in the flask (fig. 51) we may collect such an oil in the neck, since the water can escape through the tube B.
Chemico-physical Instruments
Instruments indispensable to the chemist, and which at the same time are used in Physics, are the barometer, the thermometer, and an accurate balance or pair of scales. The former instrument is sufficiently well known. As the thermometer is intended to determine the temperatures of very different substances, boiling acids, for instance, its scale must be marked directly on the glass, or else be inclosed within a glass tube. The various forms in pl. 30, figs. 37–41, are given for the purpose of introducing them into the interior of other apparatus, or in order to a more ready support.
Pl. 30, fig. 69, presents the most usual form of the ordinary Chemical Balance. The cylinder, EA, is a brass stand carrying the balance. At l is an eccentric disk, on turning which by the button k, the knife-edge on which the beam of the balance rests when in use, may be raised to prevent any injury to the delicacy of its edge. Just above l there is a small plate of ivory, graduated to degrees, for noting the movements of the tongue E. The beam of the balance is divided into ten divisions for the sake of weighing by tenths of small weights, constructed of platinum wire, and suspended from the divisions of the beam. The suspension arrangement of the scale-pans and that of the beam is shown more fully in the succeeding figures.
Pl. 30, fig. 70, represents the method of hanging the balance beam, which in the figure appears cut off at each end, so that only the two central pieces, BB, connected by the central annular body, are visible. a is a triangular prism of steel, the edge of whose lower acute angle rests on a plate of agate, b. It passes at right angles through the middle of the beam, supported at both extremities by agate plates. Both of these are carefully laid in the same plane, ee is a brass frame inclosing the body on which the agate plate rests. This frame can be raised or depressed by means of a pin connected with the excentric disk, el. When this frame is raised, it catches the very extremities of the knife edge in two opposite notches, and raises it with the beam from the agate plate. The figure represents only the anterior arrangement of the knife edge and agate plate, the posterior being precisely similar. When the knife edge is raised by the frame, ee, the two pins, hh, of an arm, gg, firmly connected with the frame, ee, catch at the same time under the scale beam, and support it still more.
Pl. 30, fig. 71. represents the suspension apparatus of the pans. The plate, m, is screwed to the end of the beam, B. At o, the pan is suspended by means of a hook in the ring of the stirrup, into which the plate, m, is continued. By means of the screw, n, the length of the beam from the knife edge to the point of the suspension may be made perfectly equal for both arms.
Pl. 30, fig. 72, is another arrangement for suspending the pans, after Hoss. The most essential part of this arrangement is a steel plate (fig. 73), provided at one end with a knife edge. This knife edge is divided into two parts by a small intersection, widening below. The hook r (fig. 72) carries in its middle a fine plate of metal, which, fitting in the intersection of the knife edge, renders a lateral displacement of the hook impossible. The parallelism of the knife edges or the equal length of the arms may be regulated by screws attached at o, p, and n.
Miscellaneous Apparatus for Special Investigations
Davy’s Safety Lamp (pl. 31, fig. 59). In mines of different kinds, in coal mines especially, a certain gas, carburetted hydrogen, often forms in considerable quantity, and this, when mixed with air, becomes highly explosive. Inflamed as it frequently is by contact with the light used by the miner, if produces the most disastrous consequences to life and property. Such accidents are prevented by the proper use of the safety lamp. The right hand figure exhibits a section of the apparatus, which consists of a frame, at the lower part of which is placed a lamp, the frame itself being completely inclosed by a wire cylinder of very fine copper gauze. On introducing this lamp into an explosive mixture, the gas penetrating the interstices of the gauze, within the inclosed space, may indeed be set on fire, but the flame cannot be communicated to the outside, owing to the rapid reduction of temperature experienced by contact with the metal.
Davy’s Apparatus for Investigating the Earths (shown in pl. 30, fig. 61), is intended specially for examining the amount of gas generated from a calcareous earth under the influence of an acid. D is a retort containing the earth, E a vessel for receiving the acid, provided beneath with a stop-cock; b is a connecting tube, to the lower end of which a contracted bladder is fastened; B is a retort filled with water, and A a graduated measure. After the earth has been introduced into D, the cock at E opened, and the acid let in on the earth, the liberated gas passes through the tube, b, into the bladder, c, expands this and thereby displaces an equal amount of water from B, which passing over to A, indicates by its level at a, the volume of gas introduced.
The Wolff Apparatus (pl. 30, figs. 56 and 57) is intended to be used for saturating liquids with soluble gases. Thus, suppose it be desired to prepare a saturated solution of chlorine in water, the generating apparatus (fig. 56) is applied to the tube, C, and the bottles, A and B, filled one third with water. On allowing the gas to enter through C, it gradually displaces all the air in the apparatus, and the water in A gradually purifies the gas of improper admixtures. The gas then passing over to the bottom of the water in B, rises through the water and is in a great measure dissolved, the water becoming gradually saturated. The excess of gas, or that which has not been dissolved, may be made to pass over into a third flask, and thence to a fourth, &c. D and F (in fig. 56) are safety tubes, through which the expansive force of the gas may be spent in driving out the water in the bottles whenever any obstruction occurs, instead of bursting the vessel. Fig. 57 exhibits the apparatus as connected with an apparatus for generating the gas.
Apparatus for making anhydrous hydrocyanic or prussic acid (pl. 30, fig. 62). In the cylinder, b, the hydrochloric acid gas is generated, which enters through the tube, a, into the porcelain tube, D, in which cyanide of mercury has been laid, and where, by the decomposition of these two compounds, produced by the heat of the coal fire, G, prussic acid is generated. In the hollow, E, of the tubular extension of D, are placed pieces of chloride of calcium and chalk, kept cool by the snow with which the vessel, ABC, is filled for the purpose of condensing any watery vapor which might form, &c. The hydrocyanic acid vapor enters the tube, F, which is surrounded by an artificial freezing mixture, as of salt, snow, and dilute sulphuric aid; by this means the volatile acid is condensed.
Pharmaceutical Steam Apparatus. Pl. 30, fig. 13, represents a quite recent form of steam apparatus very convenient for pharmaceutical purposes. The dotted box, A, is the steam boiler. Beneath it is the fire space with its door, a, and beneath this, again, the ash-hole to which the door, b, leads. From the upper plate, F, projects the matrass or alembic, C, heated by the steam of the vessel, A; G is the extremity of its neck through which the products of distillation pass. Next to this matrass are several openings on the plate into which are set the tin boxes, c, c′, c″, capable of being removed by means of their handles. They are closed by a well fitting cover, and are used for making extracts, infusions, &c., of roots, stems, leaves, &c., placed in them. The steam ascends through a tube, f, into a second and smaller vessel, D, fitted with a cover, in which are inserted arrangements, g, g′, similar to those last described, for boiling or evaporating. The vapor which collects in this boiler, and passes through the tube, h, is almost entirely condensed to water, and accumulates in the vessel, E, in which then there is always an abundant supply of distilled water so necessary for many purposes. The doors, m, m′, m″, lead to spaces which may be heated by steam, and if necessary, by a fire made in k, l. We may thus, as is readily intelligible from the figure, boil, distil, evaporate, and dry all at once by a single heating of the boiler.
Fig. 14 is a similar arrangement also explicable by the preceding description. D and C are steam boilers into which project the pots, k, k, k, and the vessels, L, M, Z. The fire space with its door is seen at B. F is the distillatory apparatus projecting by about half the length of its neck into the neck of G, in which the vapors are condensed. I is a tube from the boiler, which also goes into the cooler, H, and furnishes an uninterrupted supply of distilled water. The door, E, leads to the drying press, also heated by steam.
Fig. 15 represents the ground-plan of the fire space of the preceding apparatus. Fig. 16 is a copper vessel in the upper part of which is suspended a pan, and heated by being set in one of the openings in the upper plate of the boiler.
Fig. 17 is the porcelain pan or saucer setting in fig. 16. Fig. 18, the ring set on the copper vessel and carrying the porcelain pan. Fig. 19, a plate with four apertures, into which pots such as are seen at k, k, k, in fig. 14, may be set. This plate can be laid on an opening in the copper plate of the boiler, and the contents of the pots thus heated. Fig. 21 represents a single one of these pots.
Pharmaceutical Extract Press. Pl. 30, fig. 65, represents the hydrostatic press as given by Real. Its object is to express the juice of plants under great pressure. For this purpose such substances are introduced into the space, A, on a sieve bottom over B, and the entire space, as well as the tube, C, filled with water. This tube is made several stories high and leads to some convenient part of the house. As soon as the column of water in C is brought into communication with the water in the cylinder, A, the column of water exerts a pressure corresponding to its height, on the substances placed in A, which are therefore more completely penetrated by water. The extract obtained is removed from beneath, after a proper time, by opening the cock, d.
Pl. 30, fig. 66, is essentially the same apparatus, and is intelligible by means of the same description as in the preceding paragraph, with the variation only that a column of mercury is used instead of water. The apparatus is first filled from D, with water, the cock, e, closed, and the tube, E, filled with mercury.
Fig. 67 exhibits a press for the same purpose after Rommershausen. The substance from which the extract is to be made, must be introduced into the cylinder, A, on a sieve bottom, d, covered by a cloth, and pressed upon by a second superimposed perforated bottom, e, lightly pressed against the substance during the operation by screws passing through the cover: this is attached by clamps. The liquid by means of which the extract is to be made is poured upon the stamper, GE, by means of a measure (fig. 50), graduated on the inside. On raising the piston, E, this passes through the valve, e, into the lower space of the cylinder, and on depressing the piston, through the valve, a, into the canal, k, presses against the sieve bottom, b, and the substance between d and e, and collects above e, in the space B, from which it can be readily removed.
Fig. 68 is also an extract press, in which a pressure is exerted by means of the piston, DE, on the liquid in the space G, which covers the substance whose extract is to be obtained. B is a filtering apparatus. By opening the stopper, b, the liquid is introduced. It is removed by turning the cock c.
Pl. 30, fig. 63. An apparatus for producing small quantities of illuminating gas from various substances. The substances to be exposed to a dry distillation are placed in d. The gas passes through the connecting tube, C, into the receiver, b, which may be removed at pleasure, or from which the gas may be let off by means of a cock, e, and the tube a, or else may be burned by means of a burner set on a.
Pl. 30, fig. 64. Argand’s Oil Lamp, for drying precipitates or filters. A movable arm slides on the stand A, and can be fixed by means of a screw. This arm carries the furnace, C. B is an Argand lamp. A boiler is placed in C, having a lateral escape pipe for letting off the steam. D is a glass vessel with a rim, which rests on the edge of the boiler and closes it. In this vessel the filter to be dried is laid, and fixed by means of the piece E. When the water in the boiler has been made to boil by the lamp, the inside of the glass vessel becomes heated, and the filter dries rapidly.
The Chemical Laboratory at Giessen
The first requisite to a satisfactory pursuit of chemistry, is, in every instance, as well regulated and complete a laboratory as possible. No science is so dependent on experiment as chemistry, every fact requiring ocular demonstration. For this reason every facility rendered to chemical operations is a clear gain. Private laboratories are no guide to the general investigator, each individual arranging his own premises according to his particular line of study. Universities, however, and technical establishments, should not be without complete laboratories, in which all imaginable operations may be carried on. We have therefore concluded to present to our readers an idea of a chemical laboratory, the first one established in Europe on a large scale, and one in which, under the direction of its distinguished founder, Liebig, a host of young chemists have been trained for years past, and in which some of the most important contributions to chemical science have been prepared. Pl. 30, fig. 1, exhibits a ground-plan of this great laboratory of the University of Giessen, and under it is a perspective view of part of the analytical laboratory. The different portions of fig. 1 indicate the following parts of the building:
A, lecture room; B, analytical laboratory; C, pharmaceutical laboratory; D, instruments and library room; E, weighing room; G, steps to the second story; H, privy; KK, laboratory and cabinet of the director; L, room for preserving preparations; M, store room; N, washing room; O, room for the servants in the laboratory; P, old laboratory; Q, ante-chamber; R, yard and garden; a, black-board, capable of being raised or lowered; b, fireplace used in the lectures; c, iron door; d, closed fireplaces; e, table for nitrogen operations; f, door between laboratory and lecture room; g, potassium furnace; h, sand bath; i, work table with shelves; k, lower work table for minute operations; l, tables; m, stove; n, small water pipe; o, gutters for carrying off water; p, rain water cistern; r, pipes leading from the roof to the cistern; s, trap door for introducing coal into the coal cellar; t, door of communication; u, distilling apparatus; v, work table with shelves; v′, lower work table; w, table for using apparatus; x, fireplace with cooking vessels of different sizes; y, closed work table, for generating noxious gases; z, stove; z″, chimney; tz, two door communications; aa, book and instrument cases; bb, stove, whose pipe in winter heats the space for the water reservoir; cc, water reservoir; dd, steps to the coal cellar; ff, yard door; f′f′, window towards the laboratory; g′g′, window towards the old laboratory, P; hh, director’s fireplace; ii, passage towards the pharmaceutical laboratory and the privy.
From the bare enumeration of these different parts of this vast laboratory, it is evident that the entire course of chemical instruction is very different from the method of lectures usual in academies. The reason of this is that satisfactory and extended chemical experiments require a large stock of apparatus and great conveniences for manipulation; as, also, a large amount of time. The oft-repeated and long-continued observations required in certain experiments, render it necessary for the laboratory to be arranged for the daily residence of the students. A proper instruction in chemistry generally commences with teaching those properties of elementary bodies which are calculated to furnish distinguishing characteristics. Instruction of this kind has, however, not merely for its object the elucidation of this knowledge in the form of a certain easily understood system; it is rather intended to exhibit those principal properties of matter by experiment, and first of all to teach by what means the single elements are to be obtained, or ascertained in any given compound, however complicated. This first part of practical chemistry is known as qualitative analysis, since its object is merely to ascertain the character of the elements composing a combination. After sufficient practice of the student in qualitative analysis, instruction in quantitative analysis follows next, or the investigation of the exact amount by weight of each element or body contained in a given substance, or in any of its combinations. It is especially necessary for the student to be exercised in the most varied practical operations. A knack in handling delicate and expensive apparatus, and a constant habit of considering the most varied results which may ensue in combining single substances, must be acquired by the student in chemistry, besides the actual science which he studies.
Errata
Possible errors in the original plates compared to their descriptions.
- Plate 30:
- Two figures labeled 21 (lower is Fig. 22)
- Fig. 33 is missing
- Two figures labeled 42 (right is 41)